Abstract
The adverse effects of early-life stress are pervasive, with well-established mental and physical health consequences for exposed individuals. The impact of early adverse experiences is also highly persistent, with documented increases in risk for mental illness across the life span that are accompanied by stable alterations in neural function and hormonal responses to stress. Here, we review some of these ‘stress phenotypes’, with a focus on intermediary factors that may signal risk for long-term mental health outcomes, such as altered development of the fear regulation system. Intriguingly, recent research suggests that such stress phenotypes may persist even beyond the life span of the individuals, with consequences for their offspring and grand-offspring. Phenotypic characteristics may be transmitted to future generations via either the matriline or the patriline, a phenomenon that has been demonstrated in both human and animal studies. In this review, we highlight behavioral and epigenetic factors that may contribute to this multigenerational transmission and discuss the potential of various treatment approaches that may halt the cycle of stress phenotypes.
Introduction
It is widely recognized that early experiences often have a profound impact on individuals' functioning across their life span. For example, the median age of onset for mental health disorders as a whole is 14 years, with the majority of adult disorders emerging during childhood or adolescence (Jones 2013; Kessler et al. 2007; Lee et al. 2014). Further, certain factors within the home (such as parental mental illness, criminality, violence and neglect) are strongly associated with the onset of child/adolescent mental illness, accounting for nearly half of all childhood-onset, and nearly a third of later-onset, mental health disorders (Green et al. 2010). These data suggest that the experience of early-life adversity renders individuals prone to psychopathology across the life span. While nearly all studies on mental health (across species) focus on end-state outcomes, that is, when disordered functioning is already present (Thompson & Levitt 2010), understanding the developmental pathway toward aberrant outcomes is highly clinically relevant in order to establish early markers for identifying and treating at-risk individuals. For this reason, the focus of the first section of this article is to review some potential early development markers of risk associated with early-life stress. This is followed by a discussion of the persistence of stress-induced phenotypes across generations as well as potential mechanisms for these generational effects. Finally, given the clinically significant outcomes associated with early adversity, some potential targets for intervention are discussed.
Early adversity and the development of the individual
Animal models
The importance of animal models is accentuated in adversity research, a field that is fraught with logistical and ethical complications when considering studies of human populations. As such, animal models have been highly informative in establishing some of the immediate, short-term and long-term consequences of early adversity, and in providing insights into the developmental pathways that might increase risk for mental illness across the life span (for reviews, see Meyer & Hamel 2014; Sanchez et al. 2001). Here, we focus on work (conducted mainly in rodents) suggesting that early adversity has specific effects on the development of emotion learning systems (such as fear and extinction) that may place affected individuals at risk for dysfunction later in life.
In some early rodent studies demonstrating the effect of stress on the developing fear system, it was shown that exposure to stress or glucocorticoids within the first few weeks of postnatal life resulted in an early transition from approach to avoidance responses toward a shock-paired odor (e.g. Moriceau et al. 2009). That is, seminal studies by Sullivan demonstrated that while postnatal day (P) 12 rats avoid an odor previously paired with shock, P6 rats exhibit a paradoxical approach response toward such an odor (Sullivan 2001). However, this transition to odor-avoidance learning occurred at a younger age in infant rats exposed to a stressor (abusive care from the mother as a result of insufficient bedding) or those injected with corticosterone (Moriceau et al. 2009). We have reported other changes in learned fear behaviors in infant rats (i.e. P17) exposed to either rearing stress or glucocorticoids. Specifically, stressed or glucocorticoid-exposed infants exhibited much longer retention of learned fear responses than their same-aged, typically reared counterparts (the non-stressed peers exhibited rapid forgetting known as infantile amnesia; Callaghan & Richardson 2012; Cowan et al. 2013, also see Haroutunian & Riccio 1979). In addition to these changes in fear retention, stressed/corticosterone-exposed infant rats also exhibited greater fear relapse after extinction training, a finding normally observed only later in development (Callaghan & Richardson 2011, 2014; Cowan et al. 2013). Taken together, these outcomes suggest that the effects of adversity on the developing fear system may lead to increased risk for mental health problems via an early switch into fear systems characterized by greater emotionality (i.e. stronger aversions), longer lasting fear associations and greater relapse after attempts to inhibit the fear through a process like extinction.
The use of animal models also allows for greater exploration of the neural correlates of early adversity. There is substantial evidence that early-life stress alters the brain, both structurally (e.g. reductions in hippocampal volume) and functionally [e.g. alterations in neuroendocrine hypothalamic–pituitary–adrenal (HPA)-axis activity, neurotransmitter levels and cellular signaling; for reviews, see Gunnar & Quevedo 2007; Maccari et al. 2014; Marco et al. 2011; Tyrka et al. 2013]. As with studies of behavioral changes in the context of early adversity, the majority of research on these neural effects has been conducted in adult individuals, when pathological outcomes have already emerged. However, there is growing evidence that early-life stress affects neuroendocrine and brain functioning during development. For example, increased secretion of the stress hormone cortisol, or its rodent equivalent corticosterone, has been reported in infant rats, guinea pigs and non-human primates as a result of maternal separation (Gareau et al. 2006; Hennessy & Moorman 1989; Levine & Wiener 1988; Nishi et al. 2014). Similar elevations in the neuropeptide corticotrophin-releasing factor (CRF), which is also critically involved in the mammalian stress response, have also been observed in juvenile and adolescent primates (Coplan et al. 2005). Interestingly, elevation of CRF in adolescent bonnet macaques as a result of early exposure to stress (variable foraging demand) was associated with increased volume of the left amygdala, which in turn was associated with increased anxiety-like responses in adulthood (Coplan et al. 2014). Similarly, in the study by Moriceau et al. (2009) described earlier, the precocious development of odor-avoidance learning in stressed animals was associated with enhanced amygdala activity (measured by 2-deoxyglucose uptake), which is normally observed only in older animals (Moriceau et al. 2006). Such precocious maturation of the neural circuitry involved in emotion regulation is reminiscent of, and may underpin, the accelerated developmental trajectories observed in infant rats' learned fear behaviors (Callaghan & Richardson 2011, 2012; Cowan et al. 2013; Moriceau et al. 2009).
Humans
The current evidence supports the suggestion that neural development in children is similarly affected by early stress exposure. As one illustration, alterations in cortisol secretion are observed in children exposed to early adversity (for review, see Hunter et al. 2011), paralleling the findings of the animal literature. Although the direction of these effects in humans has been inconsistent (likely due to variation in selection criteria and experimental methodology), it is clear that early-life stress alters the neuroendocrine system in children. The precocious maturation of emotion-related neural circuitry observed in rodents is similarly reflected in studies of children using brain-imaging techniques. For example, Tottenham and colleagues have examined previously institutionalized individuals using functional magnetic resonance imaging, finding that these children exhibit a more mature pattern of prefrontal cortex–amygdala connectivity when viewing fearful faces (Gee et al. 2013). Also, in an electroencephalography study of children 2–4 years of age, McLaughlin et al. (2011) reported that institutional rearing alters the developmental trajectory of frontal asymmetry. In that study, deviations from the normal developmental trajectory predicted negative behavioral outcomes (higher internalizing symptoms) at 54 months of age.
Early adversity across generations
Considering that stress has such profound effects on the biology and behavior of exposed individuals across their life span, it is possible that these alterations may have adverse consequences for future generations (see Fig. 1 for an illustration of how this has been investigated in animals and humans).
Figure 1
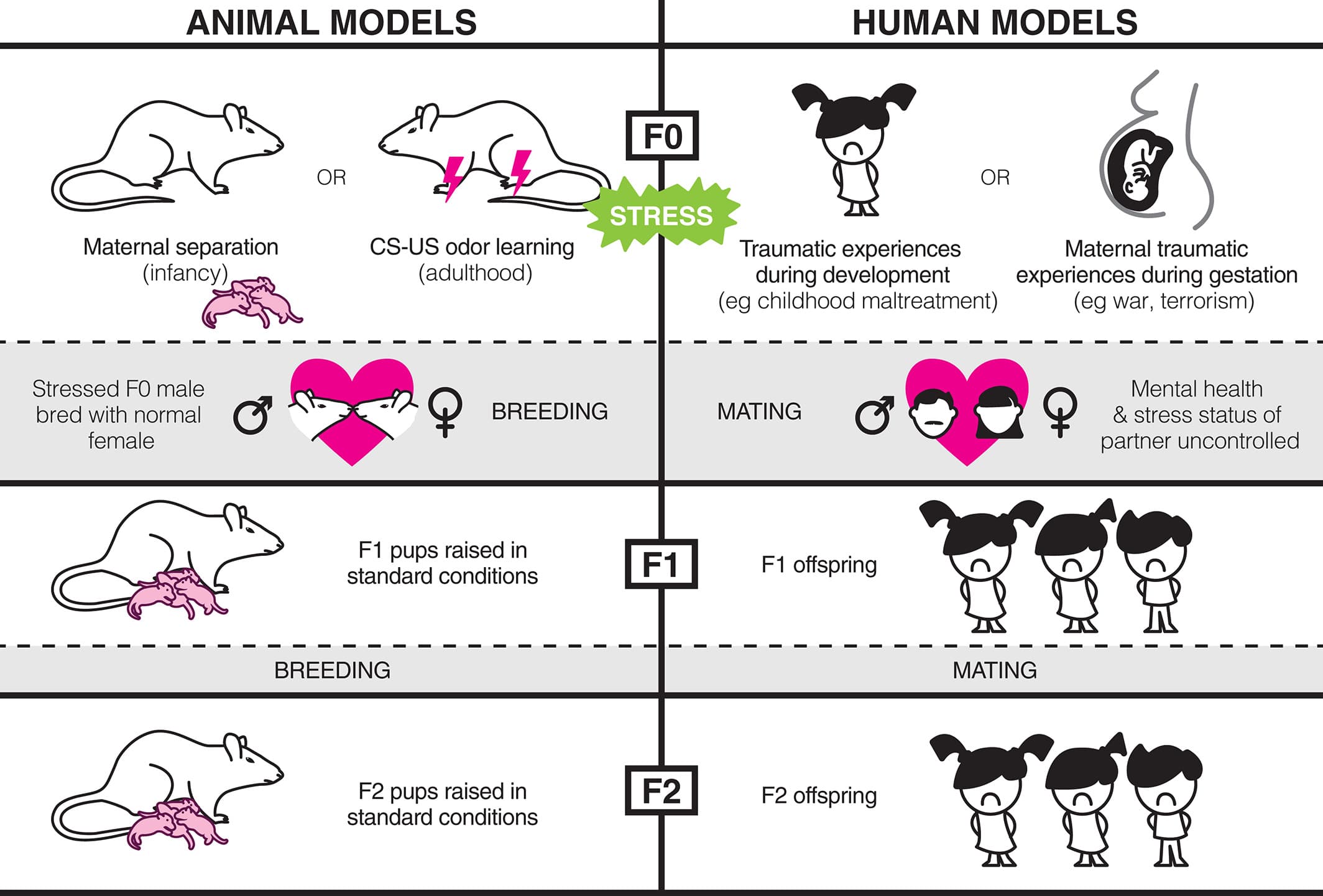
Various models of early-life stress and stressful learning experiences have been used to study the transmission of stressed phenotypes across generations in both humans and rodents. In this review, the terminology ‘F0’ is used to refer to the individual/generation directly exposed to stress. In rodent studies, F0 males are typically bred with experimentally naïve, unstressed females to produce the next generation. These offspring, known as the ‘F1’ generation, are not directly exposed to stress. Similarly, F1 males can be bred with naïve females to produce a further ‘F2’ generation. In studies of humans, the children and grandchildren of stressed individuals can be similarly labeled as the F1 and F2 generations, respectively. However, the stress exposure of mating partners and offspring is difficult to control in humans.
Animal studies
There are a growing number of studies exploring multigenerational transmission of experience-dependent characteristics in controlled laboratory conditions. In one example, Dias and Ressler (2014) used two distinctive odors (acetophenone and propanol) that elicit non-overlapping patterns of activity in the main olfactory epithelium and olfactory bulb. Adult male mice received pairings of one of these odors with an aversive shock. Subsequently, these males were mated with odor-naïve females, and their male offspring (termed the F1 generation; see Fig. 1 for further explanation of this terminology) were tested when they reached adulthood. Critically, these F1 offspring had never encountered either of these odors, nor had they ever been shocked. The results showed that the F1 males exhibited a potentiated startle response in the presence of the odor that their father had experienced with shock; further, these males had a lower detection threshold for this odor (i.e. they detected the odor at lower concentrations). Along with these behavioral effects, Dias and Ressler (2014) reported increases in the size of the glomeruli activated by the odor that the F1 male's father had experienced with shock. These effects, both behavioral and neural, were also observed when the F1 males were conceived via in vitro fertilization and persisted in the offspring of the F1 males (termed the F2 generation). Similar results were obtained when it was the mother who experienced the odor-shock pairings, an effect that persisted following cross-fostering procedures. That is, regardless of whether the odor-shock pairings were experienced by their mothers, fathers or grandfathers, subsequent generations of mice exhibited the same neuroanatomical changes and differential responses to an odor that they had never experienced prior to testing. All in all, this study provides an amazing illustration of intergenerational transmission of learned behavior and neuroanatomical structure. Multigenerational transmission of stress phenotypes has also been reported for the behavior and cortisol levels of non-human primates exposed to higher stress environments (Fairbanks et al. 2011; Kinnally et al. 2013) and for the behavior and metabolic responses of rodents exposed to maternal separation and unpredictable maternal stress (in a complex, sex-specific manner; Franklin et al. 2010; Gapp et al. 2014). It will be interesting to determine whether the early emergence of long-term, and relapse prone, fear memory in infants exposed to early-life stress (as described earlier) is also transmitted across generations.
Humans
Significant events in human history have provided researchers with natural ‘experiments’ to explore whether intergenerational transmission of stress occurs in humans. One such event is the Dutch famine (1944–1945), a 5-month period of extreme food shortage that placed significant stress on an otherwise well-nourished population (Painter et al. 2005). It was found that prenatal maternal malnutrition during later or mid-gestation resulted in physical underdevelopment (e.g. lower birth weights, heights and smaller head circumferences), whereas famine exposure during early gestation resulted in a threefold increase in coronary heart disease. Males of this generation went on to have offspring (i.e. grandchildren of the malnourished individual) who were more prone to obesity (Veenendaal et al. 2013). In addition to these findings on offspring physical health, there is also evidence that the Dutch famine impacted offspring mental health. Specifically, offspring exposed to the famine in utero had a heightened risk for schizophrenia spectrum disorders (Hoek et al. 1998), while prenatal exposure (preconception or gestational) increased symptoms of depression in adulthood (Stein et al. 2009). The intergenerational effect of trauma on offspring emotional development has also been demonstrated in studies of witnesses and survivors of natural disasters or acts of violence. For example, a study of the 1976 Tangshan earthquake in China found that prenatally exposed individuals exhibited higher rates of severe depression at 18 years of age compared with a cohort born 1 year later (and also assessed at 18 years; Watson et al. 1999), while risk for schizophrenia is consistently elevated in the offspring of mothers exposed to war during pregnancy (for review, see Babenko et al. 2015). As another example, Yehuda et al. (2005) examined women who were pregnant during the 2001 World Trade Centre attacks and later developed post-traumatic stress disorder (PTSD). Soon after the attacks these women exhibited lower salivary cortisol levels, considered a biological precursor to PTSD, when compared with a similar group of women who also witnessed the attacks during pregnancy but did not go on to develop PTSD. Importantly, the infant offspring of mothers with PTSD also exhibited low levels of salivary cortisol in the first year of life. These studies of maternal stress during gestation have provided important insights into how parental experiences persist beyond trauma exposure to impact offspring development.
The intergenerational transmission of the effects of stress is also evident when trauma exposure occurs outside of gestation. For example, offspring of combat veterans exhibit increased risk for psychological dysfunction, which appears to be exacerbated when the offspring themselves serve in combat (for a review, see Dekel & Goldblatt 2008). Adult children of Holocaust survivors born after the war, or after their parents had escaped to safety, exhibit higher lifetime prevalence rates of depression, PTSD and other anxiety disorders compared with Jewish individuals who did not have a parent who was a Holocaust survivor (Yehuda et al. 2008). In this sample, depressive disorders in adult offspring were significantly associated with paternal and/or maternal PTSD, while PTSD risk in adult offspring was uniquely associated with maternal PTSD (Yehuda et al. 2008). The presence of other anxiety disorders, such as generalized anxiety disorder and specific phobia, was elevated among all Holocaust survivor offspring regardless of parental PTSD status. Similarly, a longitudinal study conducted in post-conflict Sierra Leone found significant covariation between caregiver and child mental health, such that caregiver depression and anxiety were associated with an increase in internalizing symptoms in their adolescent offspring (Betancourt et al. 2015), while in a general population sample PTSD incidence was elevated in the offspring of women with PTSD (Roberts et al. 2012). Together, these studies highlight the inflated risk of psychiatric disorders in offspring of parents exposed to trauma. Further, they provide evidence for intergenerational transmission of stress in humans, such that individuals can acquire biological and behavioral phenotypes that match their parent's risky environment.
Potential mechanisms
The literature reviewed above demonstrates the relationship between stressful experiences (especially those occurring early in development) and later behavior, not just for directly affected individuals but also for their offspring. Exploration of the underlying mechanisms for such effects is necessary not just to deepen our understanding but also to illuminate potential avenues for intervention where the behavioral phenotype is maladaptive.
Parental behavior
One potential mechanism for transmission of parental experiences to the offspring is changes in the behavior of the affected parent. Indeed, parental styles of caregivers have been shown to be atypical post-trauma (Betancourt 2015). For example, studies of caregivers who survived the Khmer Rouge regime in Cambodia found that a role-reversing parental style mediated the relationship between maternal PTSD and offspring anxiety (Field et al. 2011, 2013). In role-reversing parenting, parents rely on children to meet their emotional needs (Macfie et al. 2005). This style of parenting is thought to interfere with a child's development of autonomy and is often evident in at-risk samples (Cummings et al. 1994). There is also some evidence that stress and trauma can result in more overtly destructive parenting approaches, such as the increased violence and poorer parental adjustment observed in families of male Vietnam veterans with PTSD (Jordan et al. 1992). Further, adult offspring of Holocaust survivors report higher levels of childhood trauma, especially emotional abuse and neglect (Yehuda et al. 2001), and the relationship between child mental health and parental war trauma in the Gaza region was found to be mediated by psychological maltreatment of the child by the parent (Palosaari et al. 2013). Maladaptive parental styles have been shown to persist into the second generation. In one study, adolescent grandchildren of Holocaust survivors perceived their parents as less accepting and overprotective in comparison to adolescents of similar cultural heritage with no family Holocaust background (Scharf 2007). More specific to early-life trauma, there is evidence that childhood abuse is associated with dysfunctional parenting practices and attitudes (Ehrensaft et al. 2015). Parents with a history of childhood abuse exhibit higher levels of emotional disengagement (i.e. lower availability, less time spent with child and higher levels of neutral affect during interactions with their child) and harsh discipline or physical punishment, as well as higher perceived ineffectiveness as a parent (Banyard 1997; DiLillo & Damashek 2003; Ehrensaft et al. 2015; Juul et al. in press). Together, these studies demonstrate that environmental insults to parents may exert enduring effects of secondary traumatization on their offspring via parental interactions, resulting in increased risk of mental health problems and difficulties in parenting their own children (Scharf 2007).
These observations in humans are supported by animal studies showing that parental care can mediate the effects of environmental adversity on offspring development. The pioneering work of Meaney highlighted the importance of maternal care for offspring development, demonstrating the links between low levels of maternal care and heightened stress reactivity, as well as the transmission of maternal behaviors across multiple generations (e.g. Francis et al. 1999; Liu et al. 1997, see also Fairbanks 1996 for review of the transmission of maternal behavior in non-human primates). In addition, maternal care has been implicated in the multigenerational effect of stressful social environments (Champagne & Meaney 2007). Specifically, female rodents exposed to social isolation showed decreased levels of maternal care toward their offspring, and this effect was transmitted to a subsequent generation. Importantly, the offspring raised by this next generation of females showed reduced exploratory behavior, an indicator of heightened anxiety-like behavior in animals.
In addition to studying the ‘vertical transmission’ of stress across multiple generations in rodents (i.e. the effects of parental stress exposure on offspring and grand-offspring), research can also be conducted within generations. This can be modeled by breeding rodent mothers multiple times following a period of stress to examine the persistent effects in caregivers (so-called ‘horizontal transmission’). Using this method, one study found a direct effect of gestational stress on maternal behavior such that stressed mothers became less nurturing than control mothers (Champagne & Meaney 2006). Those offspring raised with reduced maternal care had heightened anxiety later in adulthood. Strikingly, this style of maternal behavior and the anxious phenotype found in the offspring persisted to a subsequent set of offspring, despite the absence of any further environmental stress imposed on the mother. This line of parental transmission within generations is also currently being examined by our group using a postnatal stressor, maternal separation. The results so far suggest that the effects of maternal separation on the maturation of emotion regulation observed in infants directly exposed to that stressor are also observed in infants from a mother's subsequent litter (who were not directly exposed to any stressor; Kan et al. 2015).
Mating strategies
In rodent studies of paternal transmission of early-life stress (e.g. Dias & Ressler 2014; Franklin et al. 2010), contact between the affected parent and the offspring can be eliminated, apparently ruling out the possibility than any observed behavioral effect in the offspring is due to stress-induced changes in parental style. Nonetheless, it remains possible that there are alterations in maternal behavior that are dependent on paternal characteristics and behavior during mating (for review, see Curley et al. 2011). For instance, in a mate preference task, female rats exhibit a preference against males exposed to epigenome-modifying toxins (Crews et al. 2007). Remarkably, this preference was observed in the F3 generation (i.e. the males were not directly exposed, but rather their great-grandfathers received in utero exposure to the toxin) and prior to the onset of any disease phenotype. It has also been shown that females alter their investment in pups in response to the male's social housing experience, and that maternal investment is negatively correlated with paternal anxiety (Mashoodh et al. 2012). In other words, female rodents are able to detect altered epigenetic profiles and dysfunctional behavior in potential mating partners and adjust their behavior accordingly, being less likely to reproduce with these males and reducing investment in shared offspring when mating does occur.
Another way that mating behavior may be affected by stressful experiences is via alterations in sexual maturation. Girls exposed to early-life stress exhibit earlier onset of menarche and are more likely to reproduce at an earlier age (Chisholm et al. 2005; Quinlivan et al. 2004). Young motherhood, particularly during teenage years, is associated with poorer health outcomes for children and financial instability, with the implication that offspring are themselves exposed to a stressful early environment (Quinlivan et al. 2004). Thus, stress phenotypes may be perpetuated through the generations by a cycle of early motherhood and resource-deficient environments.
Epigenetic programming
The dynamic nature of changes to both brain and behavior in response to early-life stress, in addition to the observed transmission of these changes across generations, suggests that epigenetic mechanisms may be involved. Alterations in epigenetic regulation of gene transcription have been implicated in learning and memory, as well as the expression and multigenerational transmission of psychiatric disorders (Bale 2015; Morris & Monteggia 2014; Peña et al. 2014; Rodgers & Bale 2015). Kundakovic and Champagne (2014) provide an excellent review of potential epigenetic mechanisms for the transmission of phenotypes associated with early-life adversity, including alterations in DNA methylation, posttranslational histone modifications and non-coding RNAs. Briefly, they suggest that epigenetic changes may occur either through germline inheritance or through experience-dependent changes that are reiterated through generations via alterations in parental behavior. For example, abusive or inattentive caregiving is associated with epigenetic alterations of the neural circuitry that regulates caregiving behavior in the offspring (e.g. hypermethylation of the estrogen receptor Esr1), increasing the likelihood that offspring of abusive carers will imitate such behavior with their own offspring, thus perpetuating the cycle. Such a cyclic mechanism may at least partially account for the effects of parental behavior described above.
As noted by Szyf (2015), our current understanding of epigenetic mechanisms is still limited and there remains some skepticism regarding the stable inheritance of epigenetic marks through the germline. This skepticism is based on a long-standing principle of ‘epigenetic resetting’ whereby global demethylation during primordial germ cell differentiation was thought to lead to complete erasure of methylation patterns (Reik et al. 2001). However, emerging evidence suggests that demethylation is extensive but not complete and that experience-induced alterations in DNA methylation may indeed be inherited through the germline (for review, see Dias et al. 2015; Szyf 2015). For example, in Dias and Ressler's (2014) olfactory fear learning study, described earlier, there was significant hypomethylation of the Olfr151 genetic locus, which specifically mapped to the receptors for the conditioned odor, in the sperm of both F0 and F1 generations. In the context of early-life adversity, Franklin et al. (2010) reported increased methylation of genes coding for methyl CpG-binding protein 2 (MeCP2) and the cannabinoid receptor-1 (CB1) and decreased methylation for the genes coding for corticotrophin-releasing factor receptor 2 (CRFR2) in the sperm cells of adult males directly exposed to maternal separation. Interestingly, similar changes in methylation patterns of these genes were observed in the brains of female offspring and the sperm cells of male offspring (with the exception of CB1, which was not significantly altered in offspring sperm). Other studies (e.g. Gapp et al. 2014; Rodgers et al. 2013) have shown that paternal stress can alter sperm microRNA content and that microinjection of sperm RNA from stressed individuals into fertilized oocytes can reproduce stress phenotypes, providing another potential route for the occurrence of epigenetic inheritance through the germline.
Regardless of whether epigenetic marks are transmitted via pure germline inheritance, experience-driven patterns or some combination of the two, there are a number of potential sites that could be of functional importance for the behavioral and neural changes observed in the case of early-life adversity. Both animal and human studies have identified a broad range of genes that undergo epigenetic modification (often in a complex, brain region or tissue-specific manner) following early-life stress, including but not limited to the serotonin transporter (5-HTT; Beach et al. 2011; Kinnally et al. 2010), brain-derived neurotrophic factor (BDNF; Roth & Sweatt 2011) and MeCP2 (Franklin et al. 2010). Adversity-induced epigenetic regulation has been reviewed in detail elsewhere (e.g. Lutz & Turecki 2014), so for the purposes of this article we will limit our discussion to one potential epigenetic pathway of particular relevance for the specific behavioral effects focused on herein, that being methylation of promoter regions of the glucocorticoid receptor gene (NR3C1). Here, it is worth noting that studies of the NR3C1 gene have thus far not addressed the question of whether direct germline inheritance of epigenetic changes to this gene occurs. However, it presents as a strong candidate for further investigation. The NR3C1 gene is structurally conserved across humans and rodents, regulating the expression of glucocorticoid receptors, which provides negative feedback on the concentration of glucocorticoids to regulate their production by the HPA axis (the neuroendocrine stress response system; Suderman et al. 2012). HPA-axis dysregulation is a commonly reported consequence of early adversity that is also considered a risk factor for various forms of psychopathology, including PTSD, depression and anxiety disorders (Faravelli et al. 2012; Pesonen et al. 2010; Sanchez et al. 2001; Shea et al. 2005). It has been hypothesized that this dysregulation (which is known to persist into adulthood) is driven by changes to the DNA methylation status of promoter regions of NR3C1.
The first studies to demonstrate altered methylation of NR3C1 were conducted in infant rats exposed to differing levels of maternal care (Weaver et al. 2004). Those rats that experienced low maternal care exhibited increased DNA methylation in the exon 17 promoter region of NR3C1 in the hippocampus. In humans, hypermethylation of the exon 1F promoter region of NR3C1 (the human analog of exon 17 in rodents) has consistently been observed in individuals exposed to early-life stress and in those with associated disorders such as borderline personality disorder, major depression and PTSD (for review, see Daskalakis & Yehuda 2014). These changes are observed as early as 3–5 years of age and remain stable in adulthood (Perroud et al. 2011; Tyrka et al. 2012, 2015). Further, there is some preliminary evidence that this pattern of hypermethylation is passed on to the offspring of traumatized parents. For example, Yehuda et al. (2014) reported a complex relationship between parental PTSD and NR3C1-1F methylation, which was in turn associated with neuroendocrine responsiveness, in the offspring of Holocaust survivors. In addition, maternal war stress exposure correlated with maternal and infant NR3C1 methylation levels in a Congolese sample (Mulligan et al. 2012). Given that exposure to the glucocorticoid corticosterone is sufficient to mimic the effects of early adversity on learned fear behaviors during development in rodents (Callaghan & Richardson 2012, 2014; Moriceau et al. 2006), it is tempting to speculate that heightened glucocorticoid exposure as a result of epigenetic changes to GR expression in the offspring may contribute to the passing down of vulnerable phenotypes and subsequent risk for psychopathology.
Potential for intervention
Regardless of the mechanism of transmission, it is clear that there are altered behavioral and neural outcomes for individuals exposed to early-life adversity and that these outcomes can be passed down to future generations (see Fig. 2). In many cases, this may prepare an individual for survival in a stressful environment, but when the environment no longer matches the adaptation it can be problematic, which may explain why individuals exposed to early adversity are more vulnerable to mental health problems. Attempts to reverse or interrupt the transmission of stress-induced phenotypes across generations have been limited. Yet the nature of the stress-induced phenotype, as a response to environmental conditions, suggests that the system is inherently flexible and therefore potentially amenable to intervention.
Figure 2
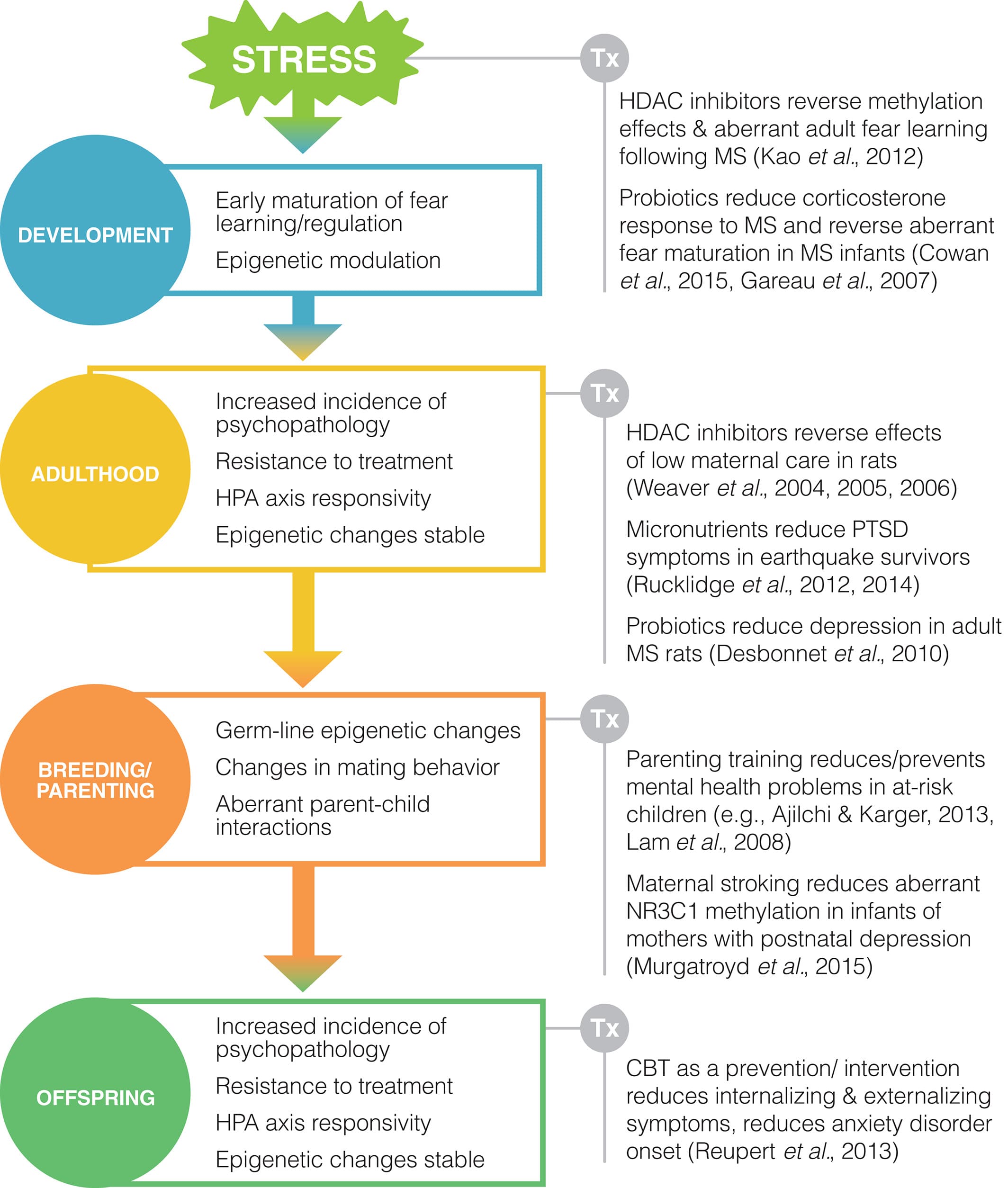
The effects of early-life stress are evident throughout an individual's life span and reverberate into the next generation. These persistent, multigenerational effects may be the result of a number of possible mechanisms, including alterations in mating behaviors, parental behaviors or epigenetic changes to the germline. Despite the deterministic implications of this evidence, the dynamic nature of these responses to environmental stimuli indicates that the system is inherently malleable. Thus, a number of potential treatment approaches for reversing early-life stress effects have been identified, including probiotics, micronutrients, epigenetic drugs and cognitive–behavioral therapies. Some of these intervention strategies have been implemented with promising results, with key intervention strategies and time points illustrated above. Abbreviations: CBT, cognitive behavioral therapy; HDAC, histone deacetylase; HPA, hypothalamic–pituitary–adrenal; MS, maternal separation; PTSD, post-traumatic stress disorder.
Traditional mental health treatments
As reviewed above, exposure to adversity during development renders individuals vulnerable to mental health issues throughout the life span. It follows that individuals with a history of early adversity are likely to benefit from effective treatments for these illnesses. Unfortunately, some concerning evidence suggests that individuals with a history of early trauma may not only exhibit a higher incidence of psychopathology but are also less likely to respond to existing treatments. Research from our laboratory shows that rodents with a history of early-life stress are more vulnerable to fear relapse following extinction (Callaghan & Richardson 2011; Cowan et al. 2013), which is in keeping with epidemiological evidence that childhood maltreatment is associated with lack of treatment response in clinical trials of psychological therapy, antidepressant medication or combined treatment for depression (Nanni et al. 2012). Similarly, both pharmacological and psychological treatments have been found to be less effective in populations exposed to early adversity across attention deficit hyperactivity disorder (ADHD; Sugimoto et al. 2015) and substance abuse (Boles et al. 2005; Sacks et al. 2008), although not in social anxiety (Bruce et al. 2013).
Turning to generational effects, to our knowledge there have been no studies directly examining the impact of early adversity on offspring treatment outcomes. However, some research has examined the impact of parental mental illness. For example, early results from an international collaborative study (the Genes for Treatment study) suggest that parental psychopathology interferes with children's recovery from anxiety disorders, predicting a significantly poorer response to treatment on follow-up (Hudson et al. 2015). On a more positive note, there are also indications that cognitive-behavioral therapy (CBT) can be effective as either a prevention or intervention technique to reduce psychological risk in the offspring of individuals with a mental illness. A number of early intervention programs have targeted these individuals with promising results (for review, see Reupert et al. 2013; Siegenthaler et al. 2012). For example, a family-based CBT program significantly reduced anxiety disorder onset in the 7- to 12-year-old offspring of anxious parents at 12-month follow-up (from 30% in the waitlist control to zero in the treatment group; Ginsburg 2009). Several studies have also found CBT-based programs to effectively reduce internalizing and externalizing symptomology and rates of illness onset in the offspring of depressed parents (Reupert et al. 2013). While these studies have generally examined relatively small samples, more definitive support for the efficacy of intervention in the F1 generation may be provided by a large-scale randomized control trial currently being conducted on the effects of a CBT intervention in children of depressed and anxious parents (described in the study protocol published by Nauta et al. 2012). Further research is also needed to identify the lifetime effectiveness of such programs and to ascertain whether effective psychosocial or pharmacological treatment of mental illness can reduce the risk of psychopathology in subsequent generations.
Parenting interventions
While therapeutic interventions that address psychopathology in affected parents and their offspring appear promising, the dysfunctional parenting practices discussed previously point to the need to consider specific targeting of parenting skills. Such parenting skills training has been shown to be effective in the treatment of children's psychopathology, particularly for childhood externalizing disorders (e.g. Triple P Positive Parenting Program and Parent–Child Interaction Therapy; for reviews, see Kazdin 1997; Thomas & Zimmer-Gembeck 2007), and also for childhood anxiety and depression (Eckshtain et al. in press; Manassis et al. 2014). In parents exhibiting psychopathology (e.g. depression, substance abuse) or exposed to various forms of stress (e.g. poverty, divorce, foster care), the evidence suggests that parenting competence is amenable to intervention (Ajilchi & Kargar 2013; Guttentag et al. 2014; Lowell et al. 2011; Oriana Linares et al. 2006; Suchman et al. 2011; Wolchik et al. 1993). Importantly, treatment-induced enhancements of parenting attitudes have been shown to be transmitted to a subsequent generation, indicating the potential generational impact of parenting interventions (Mahrer et al. 2014). Furthermore, parenting interventions have also proven to be effective at reducing or preventing negative child mental health outcomes in these at-risk samples, with reductions in child internalizing and externalizing problems reported across different forms of parental psychopathology and stress (Bywater et al. 2009; Guttentag et al. 2014; Lam et al. 2008; Lowell et al. 2011; Malmberg & Field 2013; Self-Brown et al. 2011). However, there is evidence that parenting interventions are less effective for the offspring of individuals with greater symptoms of psychopathology (e.g. Van Loon et al. 2011; Webster-Stratton & Hammond 1990, but see also Gardner et al. 2010; Timmer et al. 2011). This suggests the need to concurrently target parental psychopathology, a strategy that has been shown to be more effective in the treatment of depressed mothers and their disruptive children (Sanders & McFarland 2000).
Interestingly, an even simpler behavioral parenting technique has recently been shown to reduce epigenetic aberrations in children exposed to perinatal maternal depression (Murgatroyd et al. 2015). Specifically, Murgatroyd et al. assessed DNA methylation patterns in 181 infants born to women with varying levels of depression symptomatology. They found associations between postnatal depression and increased methylation of the NR3C1-1F promoter in infants. Strikingly, in a higher risk subgroup whose mothers reported postnatal but not prenatal depression levels above the median there was a protective effect of self-reported maternal stroking. That is, there was a negative correlation between maternal tactile stimulation of infants and the DNA methylation patterns in those infants, speaking to the importance of parent–child interactions in the modulation of epigenetic risk factors and transmission of stress phenotypes.
Targeting epigenetic changes
Psychopathological responses to early adversity are complex, with no discernible specificity of relationships between particular types of trauma and particular mental illnesses within nor across generations (Starr et al. 2014). As such, an approach that targets underlying mechanisms rather than symptomatology might prove more effective, particularly when considering long-term, heritable changes. Such an approach also fits well with the Research Domain Criteria (RDoC) framework proposed by the National Institute of Mental Health (e.g. Insel 2014). Given our discussion of the potential role of epigenetic alterations in the maintenance and transmission of stress-induced behavioral changes, targeting the epigenome seems a logical starting point. In this regard, there has been some use of epigenetic modifiers to reverse anxiety and stress-responsiveness phenotypes associated with different early experiences. Meaney and colleagues conducted the groundbreaking studies in this area (Weaver et al. 2004, 2005, 2006). They demonstrated that central infusion of either the histone deacetylase (HDAC) inhibitor trichostatin A or the methyl donor methionine could reverse the effects of low or high maternal care, respectively, on DNA methylation patterns, glucocorticoid receptor expression, HPA stress responses and behavioral expressions of depression and anxiety in adult mice.
Similar effects of epigenetic modulators have since been demonstrated in animals exposed to early stress (Kao et al. 2012). Specifically, systemic injection with an HDAC inhibitor (valproic acid) immediately prior to maternal separation and isolation reversed the effects of isolation on DNA methylation in the frontal cortex and fear-potentiated startle behavior in adulthood. This study is valuable in that it demonstrates that epigenetic treatments can confer long-lasting protection against the effects of early stress when delivered during the stressful period. However, it is not known whether these benefits might be transmitted to future generations, let alone whether such hypothetical transmission might occur through the germline or through changes to parenting behavior. Research on the use of epigenetic modifiers in humans is even more limited. However, the effect of valproic acid (a pharmacological agent widely used to treat epilepsy and bipolar disorder) on epigenetic profiles has been proposed as the mechanism for its efficacy in management of bipolar disorder (Phiel et al. 2001). Ideally, manipulation of the epigenome would be targeted at the key gene promoter regions in specific brain regions that are susceptible to stress-induced epigenetic changes (e.g. NR3C1 in the hippocampus). However, at this stage, we are far from achieving that level of specificity, suggesting that alternative treatment options need to be considered.
Micronutrients: a more palatable approach?
An alternative, and definitely more practicable, strategy for intervention involves alterations in dietary intake. As observed in the aforementioned studies of the Dutch famine, nutrition can play a key role in the overall health of individuals and their offspring. It has also been hypothesized that one driver of epigenetic changes in the context of stress is altered maternal intake of nutrients, including dietary methyl donors (Lucassen et al. 2013). Folic acid, vitamins B2, B6 and B12 are involved in DNA methylation and changes in maternal or paternal micronutrient intake can alter offspring, grand-offspring and great-grand-offspring outcomes (for review, see Vanhees et al. 2014). Further, recent evidence from studies of the effects of the Christchurch earthquake indicates that micronutrients administered in the aftermath of trauma conferred a protective effect, resulting in a long-lasting reduction in the incidence of PTSD symptoms (Rucklidge et al. 2012, 2014). It would be interesting to investigate whether this stress-protective effect also occurs in developing populations exposed to trauma.
Modification of the microbiome
Another potential source of epigenetic modifiers is the large population of commensal bacteria that reside in the gastrointestinal tract. Known collectively as the microbiome, these bacteria produce large quantities of short-chain fatty acids (e.g. butyrate, acetate and propionate), which can act as HDAC inhibitors (Licciardi et al. 2010). There is mounting evidence to suggest that the microbiome plays an important role in the expression of emotional behavior and the function of associated brain systems across species (Christian et al. 2015; Foster & McVey Neufeld 2013; Jašarević et al. 2015). For example, the composition of the microbiome has been shown to be important for the development of normal emotional behavior and stress responses in studies of both germ-free mice (raised in the absence of a microbiome; De Palma et al. 2015; Heijtz et al. 2011; Sudo et al. 2004) and mice with differing emotional and gastrointestinal profiles (Bercik et al. 2011). Further, recent evidence has shown that the gut microbiota produce metabolites with known importance for mental health (e.g. serotonin; Yano et al. 2015). Manipulation of the microbiome via administration of probiotic microorganisms, which colonize the gastrointestinal tract to bring benefits to the host organism, has been shown to improve mood and reduce anxiety in both rodents and humans (Dinan et al. 2013; Messaoudi et al. 2011; Neufeld et al. 2011; Rao et al. 2009). In addition, probiotics have been shown to alter functional network activation in healthy women completing an emotional attention task (Tillisch et al. 2013).
Importantly, there is also recent evidence to show that probiotic treatments can alter outcomes in animal models of early-life stress. Probiotic treatment of maternally separated animals has been shown to dampen corticosterone responses to separation and reduce adult expression of depressive behaviors (Desbonnet et al. 2010; Gareau et al. 2007). In addition, research from our group (Cowan et al. 2015) shows that the effects of maternal separation on development of fear-related learning and extinction are reversed by treatment with a probiotic supplement to dams' drinking water. These findings suggest that both the precocious development of fear systems and the long-term emotional dysregulation associated with early-life adversity can be reversed by targeting the microbiome. It will be important to determine the mechanism for these actions (e.g. alterations in epigenetic signals and changes in maternal behavior), as well as testing whether these results can be translated to human populations or whether such treatments would alter outcomes for the offspring of individuals exposed to early adversity.
Unfortunately, there are many places around the world where children are exposed to ‘extreme environments’ which adversely impact on physical health and brain development (Nelson 2015). Treatments that target either nutritional intake or microbial balance in the gut would be particularly appealing in such settings for a number of reasons (for further commentary, see Knight 2015). For example, individuals in these settings are often malnourished and have a number of issues in regard to gut functioning (Ashbolt 2004). Further, these sorts of interventions are relatively inexpensive to implement, in part because they do not require large numbers of individuals with advanced training. Finally, such treatment approaches are more likely to be accepted, and adhered to, than more time-demanding or invasive treatments.
Other considerations for intervention
The above discussion is by no means comprehensive in the consideration of potential interventions for the effects of early-life stress across generations. There are a variety of other promising methods that have been described in the literature, including exercise and anti-inflammatory medication (Harrison & Baune 2014). One factor that will need to be considered in future studies is the timing of the intervention, whatever it might be. Just as there are critical periods during which stress can have a particularly deleterious effect, timing is likely to play an important role in the effectiveness of treatment (Gee & Casey 2015). This will be a particularly complex process when dealing with multigenerational effects of stress, and Fig. 2 highlights some potential key points for intervention drawn from the research discussed above. Similarly, the dose and duration of exposure to both stress and treatment are likely to impact on the success of any intervention. At this stage, the parameters for successful intervention remain unclear, particularly with respect to the intergenerational impact of treatment for early stress. This leaves the door open for an exciting period in the advancement of our understanding of both the mechanisms that underpin multigenerational transmission of stress phenotypes and the clinical applications of this knowledge.