Abstract
The presence of peers increases risk taking among adolescents but not adults. We posited that the presence of peers may promote adolescent risk taking by sensitizing brain regions associated with the anticipation of potential rewards. Using fMRI, we measured brain activity in adolescents, young adults, and adults as they made decisions in a simulated driving task. Participants completed one task block while alone, and one block while their performance was observed by peers in an adjacent room. During peer observation blocks, adolescents selectively demonstrated greater activation in reward-related brain regions, including the ventral striatum and orbitofrontal cortex, and activity in these regions predicted subsequent risk taking. Brain areas associated with cognitive control were less strongly recruited by adolescents than adults, but activity in the cognitive control system did not vary with social context. Results suggest that the presence of peers increases adolescent risk taking by heightening sensitivity to the potential reward value of risky decisions.
Introduction
Teenagers are known to engage in more risky behavior than children or adults: adolescents are more likely than older or younger individuals to binge drink, smoke cigarettes, have casual sex partners, engage in violent and other criminal behavior, and to be involved in fatal or serious automobile crashes, the majority of which are caused by risky driving or driving under the influence of alcohol (Steinberg, Albert, Cauffman, Banich, Graham & Woolard, 2008). Many experts agree that these preventable behaviors present the greatest threat to the well-being of young people in industrialized societies.
Significantly, adolescent risk taking differs from that of adults in its social context as well as its incidence. One of the hallmarks of adolescent risk taking is that it is much more likely than that of adults to occur in the presence of peers, as evidenced in studies of reckless driving (Simons-Morton, Lerner & Singer, 2005), substance abuse (Chassin, Hussong & Beltran, 2009), and crime (Zimring, 1998). Relatively greater adolescent risk taking in the presence of peers could be explained simply by the fact that adolescents spend more time with friends than do adults. However, recent experimental evidence (Gardner & Steinberg, 2005; O'Brien, Albert, Chein & Steinberg, in press) indicates that adolescents’ decisions are directly influenced by the mere presence of peers. Gardner and Steinberg (2005), for instance, examined risk taking in adolescents, college undergraduates, and adults who were randomly assigned to engage in a simulated driving task alone or in the presence of two friends. They found that adolescents (and undergraduates to a lesser extent), but not adults, took a substantially greater number of risks when observed by peers.
Many research groups (Casey, Getz & Galvan, 2008; Luna, Padmanabhan & O'Hearn, 2010; Somerville, Jones & Casey, 2010; Steinberg, 2008; Van Leijenhorst, Moor, de Macks, Rombouts, Westenberg & Crone, 2010a; see also Ernst, Pine & Hardin, 2006) have posited that adolescents’ relatively greater propensity toward risky behavior reflects the joint contribution of two brain systems that affect decision-making: (i) an incentive processing system involving the ventral striatum (VS; including the nucleus accumbens, NAcc) and the orbitofrontal cortex (OFC), among other regions, which biases decision-making based on the valuation and prediction of potential rewards and punishments; and (ii) a cognitive control system, including the lateral prefrontal cortex (LPFC), which supports goal-directed decision-making by keeping impulses in check and by providing the mental machinery needed for deliberation regarding alternative choices.
Neuroimaging studies conducted in both adult and adolescent populations show that these systems contribute to decision-making in an interactive fashion, with impulsive or risky choices often coinciding with the increased engagement of incentive processing regions (Ernst, Nelson, McClure, Monk, Munson, Eshel, Zarahn, Leibenluft, Zametkin, Towbin, Blair, Charney & Pine, 2004; Hare, Camerer & Rangel, 2009; Kuhnen & Knutson, 2005; Matthews, Simmons, Lane & Paulus, 2004; McClure, Laibson, Loewenstein & Cohen, 2004) and the decreased involvement of cognitive control activity (e.g. Eshel, Nelson, Blair, Pine & Ernst, 2007; Fecteau, Knoch, Fregni, Sultani, Boggio & Pasual-Leone, 2007).
Both of these brain systems undergo considerable modification during adolescence, but on different timetables. The incentive processing system evinces dramatic remodeling in early adolescence, particularly with respect to the distribution and density of dopamine receptors (Laviola, Pascucci & Pieretti, 2001). Evidence suggests that changes in the mesocorticolimbic dopamine system result in heightened sensitivity to rewards (Spear, 2009). Regions in this brain pathway (especially the NAcc), which is implicated in the valuation and prediction of potential rewards (Breiter & Rosen, 1999; Delgado, 2007; O'Doherty, 2004; Schultz, 2010), have been found in several recent functional neuroimaging studies to show especially heightened activation during adolescence in response to reward-relevant cues and reward anticipation (Ernst, Nelson, Jazbec, McClure, Monk, Leibenluft, Blair & Pine, 2005; Ernst, Romeo & Andersen, 2009; Galvan, Hare, Parra, Penn, Voss, Glover & Casey, 2006; Geier, Terwilliger, Teslovich, Velanova & Luna, 2010; Van Leijenhorst, Zanolie, Van Meel, Westenberg, Rombouts & Crone, 2010b). Compellingly, Galvan and colleagues (Galvan, Hare, Voss, Glover & Casey, 2007) found that the degree of NAcc activity during reward anticipation was correlated with adolescents’ self-reported risk taking, providing convergent evidence that adolescents’ heightened reward sensitivity contributes to elevated real-world risk taking.
Brain regions involved in cognitive control undergo comparatively gradual and protracted maturation, involving reductions in gray matter density and increases in myelination, from preadolescence through at least the mid-20s (Asato, Terwilliger, Woo & Luna, 2010; Giedd, 2008). This maturation is thought to support improvements in executive abilities such as response inhibition (Luna ), strategic planning (Luciana, Collins, Olson & Schissel, 2009), impulse regulation (Steinberg ), and flexible rule use (Crone, Donohue, Honomichl, Wendelken & Bunge, 2006).
We propose that adolescents’ especially heightened propensity to take risks when with peers may derive from the maturational imbalance between these competing brain systems. Specifically, given the elevated reward value of peer interactions in adolescence (Blakemore, 2008; Spear, 2009), the presence of peers may sensitize the incentive processing system to respond to cues signaling the potential rewards of risky behavior. In the context of an immature capacity to down-regulate reward system outputs through control signaling, this reward-sensitive motivational state may bias adolescents’ decisions toward greater risk taking. At the neural level, the influence of peers on adolescents’ decisions may be manifested in the heightened activation of regions associated with reward valuation. Alternatively, peer presence may have a direct effect on cognitive control processes, and hence be reflected as altered activity within regions associated with impulse regulation.
To test these alternative predictions, we measured brain activity in adolescent, young adult, and adult participants as they made a series of decisions in a simulated driving game. In the game, participants rendered decisions about whether to stop at a given intersection, or to run through the intersection and chance a collision with another vehicle, with the goal of reaching the end of a track as quickly as possible to maximize a monetary reward. Risky decisions offered the potential payoff of experiencing no delay at the intersection, but also the potentially costly consequence of a crash, which added significantly to the delay. Social context was manipulated by having each participant play the game alone and while being observed by peers.
Method
Participants
Data from 40 subjects (14 adolescents – eight female, ages 14–18 years, M = 15.7, SD = 1.5; 14 young adults – seven female, ages 19–22 years, M = 20.6, SD = 0.9; and 12 adults – six female, ages 24–29 years, M = 25.6, SD = 1.9) were included in analyses. Informed consent was obtained for each subject according to a protocol approved by the institutional review boards of Princeton and Temple Universities, and each received monetary compensation for their participation.
Procedure
Task design
The Stoplight task (Figure 1) is a simple driving task in which subjects control the progression of a vehicle along a straight track, from a driver’s point of view. Subjects completed four rounds of the task; two in the first social condition and two in the second social condition. Each round used a track with 20 intersections (treated as separate trials), which took under 6 minutes to traverse (dependent on subjects’ choices and providence). At each intersection subjects rendered a decision (by button press) about whether or not to brake as the vehicle approached a changing traffic signal (which cycled from green to yellow to red). As the vehicle approached the intersection, the traffic signal turned yellow, and the subject decided whether to chance a possible crash in the intersection (GO decision), or to brake and wait for the light to return to green (STOP decision). Importantly, both the timing of the traffic signals and the probability of a crash in the associated intersections were varied so as to be unpredictable by the participant. Risk taking (i.e. not braking for the yellow light) was encouraged by offering monetary incentives for completing the course in a timely fashion. Successfully traveling through an intersection without braking saved time, whereas braking and waiting for the signal to turn green again was associated with a time delay. However, if the participant did not brake and a crash ensued, the loss of time was even greater than if the participant were to brake and wait for the light. Behavioral data from the scanner were acquired and temporally aligned to fMRI acquisitions using E-Prime (Psychology Software Tools, Pittsburgh, PA), interfaced with an LCD display, headphones, and a key-press unit. Additional task details are provided in the online Supporting Information.
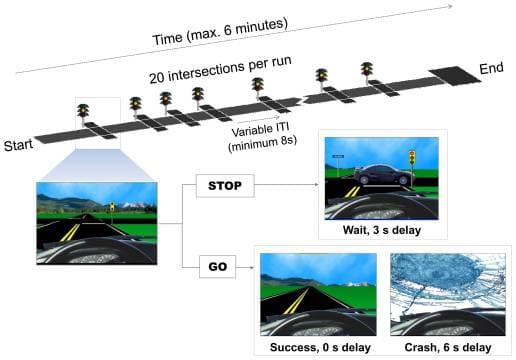
Figure 1
The Stoplight driving game. In each run of the Stoplight driving game, participants attempted to reach the end of a straight track as quickly as possible. The 20 intersections of the track were treated as separate trials, and were spaced by a variable distance (ITI). At each intersection, participants rendered a decision to either stop the vehicle (STOP) or to take a risk and run the traffic light (GO). Stops resulted in a short delay. Successful risk taking resulted in no delay. Unsuccessful risk taking resulted in a crash, and a relatively long delay. Subjects completed four runs of the task (two in each social condition).
Manipulation of social context
When reporting for the study, all participants were asked to bring two same-age (within 2 years of their own age), same-sex, friends. Social context was manipulated as a within-subjects variable, with counterbalancing for order across subjects. In an ALONE condition, participants completed the task with no observers. In a PEER condition, participants were informed that their friends were going to observe their actions from a monitor in the neighboring scanner control room. The change of social context was a surprise manipulation.1 In the break prior to the onset of the PEER condition, and in the breaks following each subsequent functional scan of this condition, the peers were asked to communicate with the scanned subject via the scanner's intercom system. In order for the interaction to be ecologically valid, the peers were permitted to speak authentically while informing the scanned participant of their presence, demonstrating their ability to observe task performance on the monitor, and communicating that they had made predictions about the scanned participant’s pending performance. The peers were carefully instructed to make these specific points during the interaction, and to avoid comments that might explicitly or intentionally bias behavior.
Self-report questionnaires
Following the fMRI session, subjects were also asked to complete a series of self-report questionnaires. Scores obtained from these questionnaires were used to assess individual differences in impulsivity (shortened form of the Barratt Impulsiveness Scale, Version 1; Patton, Stanford & Barratt, 1995), sensation seeking (assessed using a subset of six items from the Zuckerman Sensation Seeking Scale; Zuckerman, Eysenck & Eysenck, 1978), and resistance to peer influence (assessed using the RPI scale; Steinberg & Monahan, 2007). Self-report data from individual questionnaires were missing or incomplete for some subjects.
fMRI Data Acquisition
Subjects were scanned using a head-only 3 Tesla Siemens (Erlangen, Germany) Allegra magnet located at Princeton University. A T1-weighted magnetization-prepared rapid-acquisition gradient echo (MPRAGE) scan collected in the sagittal plane provided high resolution (1mm3) 3D structural imaging of the whole head, for use in subject coregistration. Each functional scan of Stoplight task performance included 195 acquisitions collected with a whole brain T2*-weighted echoplanar imaging (EPI) sequence (TR = 2.0s, TE = 30ms, flip = 70°, 33 slices, 3 mm slice thickness with 1 mm gap, 3 × 3mm in-plane resolution).2
fMRI Data Analysis
fMRI data analysis was performed using AFNI (Cox, 1996). Preprocessing of functional data consisted of several stages, beginning with a six-parameter rigid-body motion correction in three dimensions, and coregistration of the corrected functional and structural images. FMRI acquisitions requiring motion correction greater than 4 mm of translation or 4 degrees of rotation in any dimension were censored out of the dataset. Data were then interpolated to correct for slice acquisition order effects, normalized to Montreal Neurological Institute (MNI) coordinates, and smoothed with a 6 mm full-width at half maximum Gaussian kernel. The corrected fMRI data from each individual subject were analyzed in an event-related fashion using a general linear model (GLM). To explore the neurobiological correlates of age differences in the impact of social context on risky decision-making, we examined BOLD fMRI activity associated with the decision-making period of the Stoplight task.3 Specifically, event-related time-series indexing the moment when the traffic signal at each intersection cycled from green to yellow were created, and the resulting time-series were convolved with a canonical model of the hemodynamic response function (Boynton, Engel, Glover & Heeger, 1996). These event-related time-series were combined according to the social context in which they occurred to form separate PEER and ALONE condition regressors. These two regressors were entered into a single GLM equation to identify voxels exhibiting fMRI signal changes at the point of decision-making in each social context. The GLM equation also included covariates of non-interest that captured noise due to variation in run-based means, linear and quadratic scanner drift, and estimated motion. To further reduce noise, voxel-wise parameter estimates obtained from individual subjects were further subjected to outlier detection (> 2.5 SD) and removal prior to group testing. The voxel-wise parameter estimates (beta coefficients) obtained from individual subjects were entered into a group random-effects analysis in order to identify regions exhibiting main and interactive effects for age and social context. These group analyses were based on a two-way repeated measures ANOVA, treating age group as a between-subjects factor and social context as a within-subjects (repeated) factor. Additional planned, pair-wise contrasts were conducted to further clarify the differences driving significant main and interactive effects. Group-wise statistical maps obtained for all effects were constrained to an anatomical mask including cortical and subcortical gray matter, and were corrected for multiple comparisons using a voxel-wise probability threshold (p < .005) and contiguity requirement (seven adjacent voxels) that resulted in a family-wise error (FWE) rate below .05, based on Monte Carlo simulations.
Results
Behavioral results
We assessed behavioral sensitivity to social context by contrasting decision-making in the PEER and ALONE conditions. As in Gardner and Steinberg (2005), we found that adolescents and older participants behaved comparably when tested alone, but that performance in the adolescent group was sensitive to social context. Although the age by social context interaction did not reach statistical significance at this sample size [F(2, 38) = 2.66, p = .084), only adolescents took significantly more risks when observed by peers than when alone (Figure 2), as evidenced by a significantly increased number of GO decisions [t(13) = 2.16, p = .025, one-tailed] and subsequent crashes [t(13) = 4.06, p < .001, one-tailed]. Additional behavioral results are provided in the online Supporting Information.
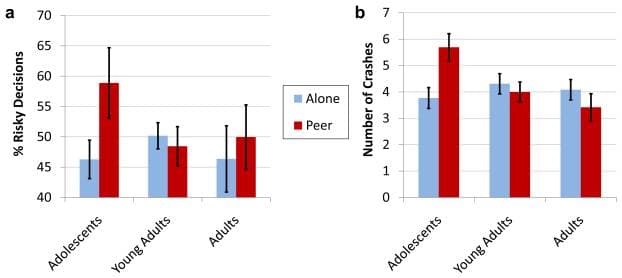
Figure 2
Stoplight task performance. Mean (a) percentage of risky decisions and (b) number of crashes for adolescent, young adult, and adult participants when playing the Stoplight task alone and with a peer audience. Error bars indicate standard error of the mean.
In order to assess the construct validity for the scanner implementation of the Stoplight task, we examined correlations between task performance and subject’s self-report responses. Variation in Stoplight performance may reflect inter-subject differences in both sensation seeking (i.e. by impelling a player to take risks) and inhibitory control (i.e. by moderating a player’s ability to regulate braking). However, a previous large-scale individual differences study found that variation in Stoplight task performance was significantly predicted by self-reported sensation seeking, but not self-reported impulsivity (Steinberg ). Despite the much smaller sample size, the present results replicate these earlier findings. As in the larger-scale study, we conducted a regression analysis in which self-report measures of sensation seeking and impulsivity were entered as simultaneous predictors of risky driving in the Stoplight task (ALONE condition), and found that behavior was significantly predicted by sensation seeking (s = .438, t = 2.40, p = .024), but not impulsivity (s = −.009, t = .049, ns). These findings provide further validation for the scanner implementation of the task, and suggest that differences in individuals’ reward- or thrill-seeking biases have an especially strong influence on task performance.
fMRI results
Regions exhibiting significant main and interactive effects of age and social context are shown in Table 1. In light of our neurodevelopmental framework, we focused subsequent planned analyses on regions showing either a main effect of age, or an age by social context interaction. Pair-wise contrasts between age groups (adolescents vs. young adults, adolescents vs. adults, young adults vs. adults) indicated that for all of the regions exhibiting a main effect of age, the effect was driven by significantly stronger engagement in adults relative to adolescents (no significant clusters were present for the other pair-wise comparisons). Notably, this pattern of greater regional activation for adult participants was observed in several left LPFC sites (Figure 3a), with young adults demonstrating an intermediate (not significantly different from either adolescents or adults) level of LPFC engagement (Figure 3c, left).
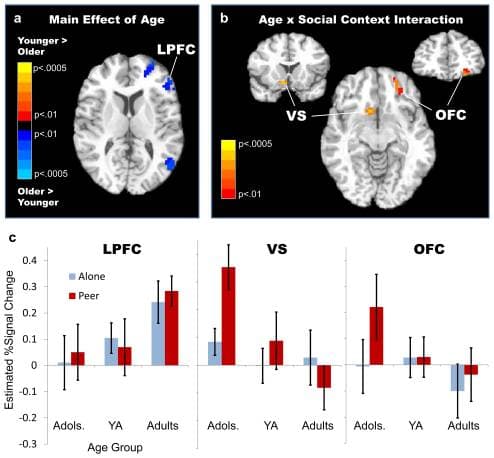
Figure 3
Regions showing a main effect of age and an age by social condition interaction. (a) Regions showing a main effect of age, including the left lateral prefrontal cortex (LPFC, MNI peak coordinates: x = –46, y = 11, z = 26, BA 46), (b) Regions exhibiting an age × social condition interaction, including the right ventral striatum (VS, MNI peak coordinates: x = 9, y = 12, z = −8) and left orbitofrontal cortex (OFC, MNI peak coordinates: x = −22, y = 47, z = −10), and (c) Mean estimated BOLD signal change (beta coefficients) from the four peak voxels of the LPFC (left), VS (middle), and OFC (right) in adolescents (adols.), young adults (YA), and adults under ALONE and PEER conditions. Error bars indicate standard error of the mean. Brain images are shown by radiological convention (left on right), and thresholded at p < .01 for presentation purposes.
Table 1
Regions showing significant (FWE < .05) main and interactive effects of age and social condition in association with Stoplight Task decision-making
Region | BA | x | y | z | mm 3 | |
Main Effect of Age | ||||||
Adults > Adols. | ||||||
L Middle Frontal | 6 | −31 | 5 | 56 | 1404 | |
L Inferior Parietal | 40 | −52 | −37 | 41 | 243 | |
L Middle Frontal ( LPFC) | 46 | −46 | 11 | 26 | 540 | |
L Middle Temporal | 19 | −53 | −62 | 15 | 972 | |
L Middle Frontal | 10 | −25 | 56 | 8 | 351 | |
L Fusiform | 37 | −52 | −55 | −19 | 540 | |
n.s. for all other pair-wise contrasts | ||||||
Main Effect of Social Context | ||||||
Peer > Alone | ||||||
L Cuneus/Sup. Occipital | 19 | −22 | −82 | 32 | 297 | |
Alone > Peer | ||||||
Precuneus | 7 | −2 | −58 | 32 | 891 | |
L Superior Frontal | 9/8 | −10 | 53 | 38 | 540 | |
Cingulate | 24/23 | −1 | −22 | 35 | 351 | |
R Middle Temporal | 21/38 | 59 | 8 | −16 | 189 | |
Interaction of Age × Social Context | ||||||
Ventral Striatum ( VS ) | 9 | 12 | −8 | 297 | ||
Mid. Orbitofrontal ( OFC) | 11 | −22 | 47 | −10 | 459 |
BA = Brodmann's Areax,y,z = MNI coordinates
Consistent with the prediction that peer presence especially sensitizes incentive processing in adolescents, significant age by social context interactions were found selectively in the VS and OFC – regions known to be involved in reward prediction and valuation (Figures 3b and 3c). Planned within-group contrasts of PEER versus ALONE condition activity indicated significantly greater PEER condition activation of the VS and OFC among adolescents, but not in the other two groups. Furthermore, direct comparison of age groups within each social condition indicated greater activation in these incentive processing regions among adolescents, relative to adults, in the PEER but not the ALONE condition. These results were further corroborated by an independent voxel-wise test for regions showing a correlation between age and the magnitude of the neural peer effect (i.e. the difference between activity in the PEER and ALONE conditions), which indicated significant inverse correlations [r(38) < −.40, p < .01] between age and context-dependent activation in both the VS and OFC.
We additionally examined trial-based variation in the magnitude of decision-related activity to determine if it could explain the riskiness of the subsequent decision (GO vs. STOP). Specifically, we treated the obtained LPFC, VS, and OFC clusters as regions-of-interest (ROI) and compared activity in these regions during GO versus STOP trials (collapsing across social context). Among adolescent subjects, greater activity in both the VS (Figure 4a) and OFC was associated with risky decision-making, as indicated by significantly increased activity in these regions for GO relative to STOP trials. No decision-dependent differences were found in these regions for older age groups, and activity in the LPFC was statistically equivalent for GO and STOP trials regardless of age (additional detailing of activation in the VS, OFC, and LPFC clusters is provided with the online Supporting Information).Figure 4Activity in the right ventral striatum (VS). Estimated activity was extracted from an average of the four peak voxels in the VS ROI. (a) Estimated VS activity for all GO and STOP trials in adolescents (adols.), young adults (YA), and adults. Significantly different VS activity for GO relative to STOP trials was found for only the adolescents. Error bars indicate standard error of the mean. (b) Scatterplot of activity in the VS indicating an inverse linear correlation between self-reported resistance to peer influence (RPI) and the neural peer effect (βpeer − βalone).
Additional evidence of the relationship between anticipatory incentive processing and the peer influence on adolescent risk taking was obtained by testing the correlations between activity in each ROI and self-reported sensation seeking, impulsivity, and resistance to peer influence. Whereas individual differences in self-reported sensation seeking and impulsivity were not predicted by the activity patterns observed in any ROI, self-reported resistance to peer influence (RPI) correlated significantly with individual variation in the neural peer effect (Peer vs. Alone) exhibited by the VS [r(28) = −.54, p < .01; Figure 4b]. This relationship remained significant even when age was controlled [r(28) = −.51, p < .01]. In other words, participants’ perception of their susceptibility to peer influence was predicted by the sensitivity of VS output to social context, and this relationship was not diminished when the relationship between age and these two variables was taken into account.
Discussion
As expected, we found that adolescents, but not adults, exhibited increased risk taking when observed by their peers. This behavioral outcome replicates past findings (Gardner & Steinberg, 2005) despite the unique manipulation of social context that was required to accommodate the fMRI environment. Indeed, these behavioral findings have intrinsic value (even without the complementary fMRI results) in further explicating the origins of the peer effect on adolescent decision-making. Since peers were located in a separate room and were prevented from interacting with participants during the decision-making task, adolescents’ heightened inclination to take risks when watched by their friends cannot be explained, at least in this study, by greater explicit encouragement from their peers to engage in risky behavior. In other words, the observed peer effect was not due to overt ‘peer pressure’.
We posited that the risk-promoting effect of peer presence on adolescent decision-making could arise from a neural ‘vulnerability’ that emerges due to the discordant maturation of the brain systems that support decision-making. Accordingly, we sought to determine whether the peer effect might result from alterations to the activity of neural systems underlying cognitive control, incentive processing, or both. As in several prior studies of age-dependent effects on reward processing, we observed differential anticipatory activity in the VS and OFC across age groups. However, our key finding is that this age difference in reward system activity was dependent on social context, consistent with the hypothesis that the presence of peers differentially sensitizes adolescents to the reward value of risky choices. Specifically, relative to adults, adolescents demonstrated significantly greater activation of VS and OFC as they rendered decisions about risk, but only when they were aware that their friends were watching them. Meanwhile, adults showed no differences in the activation of these regions as a function of social context. Indeed, among adult participants, activity in these known reward-sensitive regions was not above baseline in either social context, and in fact skewed negatively in the PEER condition. This pattern of activity may simply indicate that older participants did not perceive task events as potentially rewarding, despite the contingency to a later monetary incentive. However, an alternative explanation is provided within our neurodevelopmental account, which posits that the presence of peers is not rewarding for adults, and that adults are better able to recruit the LPFC to actively suppress reward system outputs as they enact strategic decision-making.
Consistent with this account, and with previously observed differences between adolescents and adults in the recruitment of the cognitive control system during decision-making, we found that adults engaged multiple LPFC sites more robustly than did adolescents when making decisions in the Stoplight task. Importantly, this age difference was evinced regardless of the presence of peers (i.e. it did not interact with social context). These results indicate that while there are age-dependent differences in the recruitment of cognitive control processes, the behavior of this system is insensitive to the manipulation of peer context, and does not principally account for the peer influence on risk taking seen during adolescence but not adulthood. Given that LPFC activation has been associated with calculation of outcome probabilities in support of strategic approaches to risky decision-making (Heekeren, Marrett, Ruff, Bandettini & Ungerleider, 2006; Tobler, Christopoulos, O'Doherty, Dolan & Schultz, 2008; Venkatraman, Payne, Bettman, Luce & Huettel, 2009), we speculate that the stronger activation of LPFC by adults in our study corresponds to a greater reliance on a deliberative strategy to guide decision-making.
Age differences in the context-dependent activation of VS and OFC suggest that adolescents’ heightened susceptibility to peer influence is due more to the impact of peers on incentive processing than on cognitive control. Evidence that VS and OFC activation tracked with the riskiness of subsequent decision-making (GO vs. STOP) in the driving task further suggests that the reward sensitization effect of peer presence translates into an observable increase in risky behavior. Moreover, the significant negative correlation between VS sensitivity to social context and self-reported resistance to peer influence (RPI) indicates that the brain – behavior link observed in our fMRI paradigm reflects an ecologically relevant process that is implicated in risky decision-making in the real world.
Importantly, the social influence on adolescent decision-making was indexed as a phasic interaction between social information processing and reward-related processing, and not as a state-based effect of social context. Accordingly, the significant age by social context interactions are not readily explained as simple age-related differences in the degree of social information processing, which would more likely be reflected as sustained activation differences between PEER and ALONE runs. Rather, these interactions were manifest in the differential magnitude of the transient BOLD response associated with the moment of decision-making, and the magnitude of this transient response forecast subsequent decision-making. It is thus unsurprising that other known components of the social brain (Adolphs, 2003) (e.g. ventromedial PFC, superior temporal gyrus) were not implicated in the interaction. There is evidence that changes in the density and distribution of receptors for dopamine and oxytocin within regions critical to incentive processing take place around the time of puberty (Spear, 2009), and that these changes coincide with a dramatic elevation in the salience of peer interactions (Csikszentmihalyi, Larson & Prescott, 1977). Relative to children and adults, adolescents show heightened activation within incentive processing regions in response to a variety of social stimuli, such as facial expressions and social feedback (Blakemore, 2008). Given the elevated salience and reward value of peer interaction in adolescence (Spear, 2009), awareness of peer observation could act as a tonic stimulus to incentive processing circuits, which are subsequently sensitized to respond to the potential rewards of risky choices.
The present work supports this speculation, and represents an important step toward characterizing the neurobiological mechanisms that are instrumental in adolescent risk taking. However, our task design precludes delineation of the specific reward processes that might be affected by peer context (e.g. reward valuation vs. prediction). Recent work dissociating the functions of ‘decision value’ versus ‘prediction error’ calculations in adolescents’ reward seeking behavior (Cohen, Asarnow, Sabb, Bilder, Bookheimer, Knowlton & Poldrack, 2010) suggests that it may be possible to separately evaluate these reward-specific processes, and we believe this approach could be usefully extended into future investigations of the peer effect. Moreover, it should be noted that our risk-taking task necessarily confounded decision-making and reward-anticipation processes. We accordingly acknowledge that although the primary effect of interest (the age by social context interaction) was observed in brain areas with known involvement in reward processing, we cannot completely rule out alternative explanations. For instance, increased adolescent VS output in the peer condition could reflect an impact of social context on uncertainty during decision-making (rather than on reward processing per se), greater vulnerability to distraction (of any kind) among this age group, or simply differences in motoric output (number of button presses) across age groups and social context. We believe, however, that the involvement of these alternative processes would have been signaled by additional activity differences in brain areas known to be influenced by these factors, and no such patterns were evident.
The present findings expand our understanding of the mechanisms through which teenagers are influenced by their friends to engage in health-compromising behavior, and thus have the potential to inform strategies for intervening to reduce adolescent risk taking. The results provide evidence indicating that awareness of peers selectively amplifies activity in the adolescent brain’s incentive processing system, which in turn influences subsequent decisions about risk. These alterations in brain activity occur even in the absence of direct interactions between the adolescent participants and their peers, and are thus not easily explained as the result of explicit peer pressure to engage in risky activity. The findings extend recent efforts to connect adolescent decision-making to patterns of brain maturation by showing how the neural substrates of adolescent decision-making can be altered by the context in which it occurs. Investigation of the neural pathways through which these contextual factors influence behavior may provide the cornerstone needed to link existing behavioral and neuroscientific findings, and to ultimately understand the decisions that adolescents make when faced with potentially risky choices.