Abstract
Sensation seeking is a personality construct associated with an increased propensity for engaging in risk-taking. Associations with deleterious outcomes ranging from mental health impairments to increased mortality rates highlight important public health concerns related to this construct. Although some have suggested that increased neural responsivity to reward within the ventral striatum (e.g., nucleus accumbens) may drive sensation seeking behaviors, few studies have examined the neural mechanisms associated with stable individual differences in sensation seeking across development. To address this issue, the current study used functional magnetic resonance imaging to examine the association between neural responding to reward and stable patterns of sensation seeking across a three-year follow-up period among healthy adolescents and young adults (N = 139). Results indicated that during early adolescence (~ages 10–12), increased reactivity to reward within the nucleus accumbens (NAcc) was associated with lower levels of sensation seeking across a three-year follow-up. In middle adolescence (~ages 12–16), there was no evidence of a relationship between NAcc reactivity and sensation seeking. However, during the transition from late adolescence into adulthood (~ages 17–25), heightened reward-related reactivity in the NAcc was linked to increased sensation seeking. Findings suggest that the neural mechanisms underlying individual differences in trait-like levels of sensation seeking change from early to late adolescence.
1. Introduction
Sensation seeking is a personality trait associated with a propensity to seek out novel, highly stimulating experiences, and the willingness to take risks in pursuit of these experiences (M. Zuckerman & Kuhlman, 2000). Compared to low sensation seekers, individuals high in sensation seeking ascribe fewer consequences and less danger to their involvement in risky activities (Horvath & Zuckerman, 1993; M. Zuckerman & Kuhlman, 2000). In some instances, such as engagement in some acts of courage and bravery, evidence suggests that sensation-seeking may play an important role in promoting these functional behaviors (Neria, Solomon, Ginzburg, & Dekel, 2000). However, an extensive body of research has also documented links between sensation seeking and an array of deleterious outcomes such as severe substance use impairments (Ball, Carroll, & Rounsaville, 1994), comorbid psychopathology (Slutske et al., 2002), poorer treatment outcomes (Staiger, Kambouropoulos, & Dawe, 2007), and increased rates of physical injury and mortality (Cservenka, Herting, Seghete, Hudson, & Nagel, 2013). The high societal costs attributed to this facet of personality have led to its recognition as an important public health concern (Steinberg, 2008), and further underscores the need to improve our understanding of its development.
Sensation seeking has a genetic basis (Joseph, Liu, Jiang, Lynam, & Kelly, 2009; Terracciano et al., 2011), with heritability estimates generally ranging from 40% to 60% (Stoel, De Geus, & Boomsma, 2006). In addition, shared genetic factors underlie sensation seeking and externalizing psychopathology such as substance dependence and conduct disorder (Harden & Mann, 2015; Mann et al., 2016). Changes in the incentive processing system, particularly increased responsivity to reward, likely plays a key role in explaining individual differences in sensation seeking (Dalley et al., 2007; Harden & Mann, 2015; M. Zuckerman, 1984).
Research investigating neural sensitivity to rewards as a marker of sensation seeking has largely focused on key regions in the mesolimbic dopamine (DA) system, particularly the nucleus accumbens (NAcc) (Bornovalova et al., 2009; Ikemoto & Panksepp, 1999). Findings across basic and clinical science indicate that the DA system undergoes dramatic alterations beginning around the onset of puberty (Galvan, 2010; Wahlstrom, Collins, White, & Luciana, 2010). For example, animal research has shown that DA levels in the striatum increase during adolescence (Andersen, Dumont, & Teicher, 1997) and that the firing rate of DA neurons increase at the beginning in adolescence, peak during late adolescence, and decline into adulthood (McCutcheon & Marinelli, 2009). Similarly, research in humans suggests that compared to other age groups, adolescents appear to experience particularly exaggerated reactivity to rewards in the NAcc (Galvan, 2010; C. F. Geier, Terwilliger, Teslovich, Velanova, & Luna, 2010; Luciana, Wahlstrom, Porter, & Collins, 2012; Beatriz Luna, Paulsen, Padmanabhan, & Geier, 2013; Padmanabhan, Geier, Ordaz, Teslovich, & Luna, 2011; Paulsen, Hallquist, Geier, & Luna, 2015), a region that is a principal target of DA neurons (Haber & Knutson, 2010; Ikemoto & Panksepp, 1999).
Accumulating evidence suggests NAcc response to reward may be related to increased sensation seeking during late adolescence and adulthood. There is a particular interest in delineating this association in adolescence given that sensation seeking is often found to peak at this time (Steinberg, 2008). Initial studies report somewhat discordant findings. Using a monetary incentive delay task (MID) among a sample of 26 adolescents (ages 12–16), Bjork, Knutson, and Hommer (2008), found a positive relationship between NAcc activation to anticipated rewards and sensation seeking (p < .05). Similarly, using a rewarded delayed response task with children (ages 7–11) and adolescents (ages 13–17), Galvan, Hare, Voss, Glover, and Casey (2007) found an association between NAcc response and risk perception in adolescents but not in children. Finally, Cservenka et al. (2013) using a modified version of the Wheel of Fortune task found no differences in NAcc activity to reward feedback between High (n = 27) and Low (n = 27) sensation seeking adolescents (mean age = 13.94, SD = 1.05). In sum, although a direct relationship between NAcc reward response and sensation seeking has yet to be clearly established; if such a relationship does exist, it appears this association may vary by both task and age.
Emerging literature indicates that the role of the mesolimbic system may change through development as several lines of evidence suggest that there may be a peak in dopamine availability (Padmanabhan & Luna, 2014; Wahlstrom et al., 2010). fMRI studies have reported that activity in NAcc may be different in children than at older ages. Using a rewarded cognitive control task, Paulsen et al. (2015) found increased NAcc activation in children even in the absence of rewards was associated with better cognitive control, while greater reliance on NAcc later in development was associated with decreased control. Continued predominant reliance on NAcc at later ages may reflect persistent immaturities in transitioning to reliance on cognitive control regions. However, probing the predictive significance of neuroimaging data on later outcomes has been difficult. Typically neuroimaging analyses consider brain activation as the outcome of interest (i.e., dependent variable). Recently, more studies have begun to implement a brain-as-predictor approach, wherein brain activation is modeled as an independent variable that can be used to predict longitudinal outcomes. In this approach, regions of interest are defined a priori based on strong empirical and theoretical evidence. Next, neural activation in the a priori specified region or regions is measured, followed by the collection of longitudinal data on the outcome of interest (see Berkman & Falk, 2013 for a more detailed review). In this manner, an analytic model can be specified to probe the predictive validity of the hypothesized brain region to predict the longitudinal outcome data. This methodology provides a more direct manner for testing theoretical models, that can inform ecologically validity and long-term outcomes (Berkman & Falk, 2013; Gonzalez & Berman, 2010).
Current Study
The current study investigated activation in NAcc, given its well established role in reward processing, as a biomarker of sensation seeking across development. Reward sensitivity was assessed in 139 participants ranging in age from 10- to 25-years-old using an incentivized antisaccade task. Sensation seeking was assessed at the time of the scan and then at two follow-ups conducted one-and-a-half years apart. Reward sensitivity was specified as a predictor of trait sensation seeking using a latent variable modeling framework. Developmental effects were investigated by examining the moderating influence of participant’s age on the association between reward related brain activity and sensation seeking. Given previous evidence suggesting that the association between NAcc activation and markers of risk taking may differ with development (Galvan et al., 2007; Paulsen et al., 2015), we hypothesized that reward activation in the NAcc would similarly show a different association with sensation seeking across age.
2. Materials and Methods
2.1 Participants
Data was collected as part of an ongoing neuroimaging study focused on characterizing the neural basis of cognitive control across development. Participants included a community based sample of healthy volunteers from Pittsburgh and surrounding areas who underwent behavioral and neuroimaging testing. Volunteers were native English speakers screened by phone to ensure no neurological, psychiatric, or eye movement problems, a negative history of medications known to affect brain function or eye movements, and no first degree relatives with schizophrenia or bipolar disorder. In addition, all participants had far visual acuity of at least 20/40 (corrected or uncorrected) and were screened for non-removable metal on the body, claustrophobia, and weight under 300 pounds to ensure scanner eligibility.
This study initially included 171 participants, with the sample being further refined by only including cases which met the following inclusionary criteria: runs with fewer than 15% volumes having greater than .3 mm head motion between volumes; visits sharing 90% of whole-brain coverage with all other subjects; subject visits with greater than 50% accuracy in antisaccade performance per condition; visits with 20 or more correct antisaccade trials per condition; and subjects having available data on the sensation seeking measure. This resulted in a final sample of size of 139 participants (76 females) ranging in age from 10- to 25-years-old (M=16.43, SD=3.77). Participants were assessed for IQ using the Wechsler Abbreviated Scale of Intelligence (WASI; Wechsler, 1999), and demonstrated scores in the normal range (M=110.99, SD=11.64). There were no associations found between any study control variables (i.e., gender, IQ, ethnicity), however, participant’s gender was related to the study outcome (B = −.21, p < .05) and therefore controlled for in all study analyses. No differences were found between participants included in this study’s final sample when compared to those meeting exclusionary criteria in terms of IQ (t(169) = −1.13, p > .05), gender (χ2(1) = .973, p > .05), ethnicity (χ2 = 1.67(1), p > .05), or scores on the sensation seeking measure (t(169) = −.244, p > .05). However, on average, excluded participants (M = 14.73, SD = 3.39) were younger than those included in the final study sample (M = 16.44, SD = 3.75; t = −2.60(169), p < .05). All experimental procedures in this study were approved by the Institutional Review Board at the University of Pittsburgh. Participants were paid US $75 for their participation in the study in addition to any performance-related earnings.
2.2 Procedures
Immediately prior to scanning, participants were asked to choose a reward to work towards based on their performance, which included a series of gift cards to retail locations or $25 cash. Participants were then instructed to rate how ‘valuable’ (7-point Likert scale) they considered their chosen reward and to indicate at least one item they might purchase with this compensation as a means to increase the salience of the reward. Prior to each trial, participants were informed whether they could win, lose, or neither win nor lose points on the upcoming trial by a set of colored bars. Potential reward trials were indicated by green filled bars above a central cross-hair and potential loss trials were indicated by red filled bars located below the central fixation. Neutral trials, where the subject’s performance would not affect the number of points they earned, were indicated by unfilled bars.
Subjects were informed that the number of filled bars would correlate with the number of points at stake for the upcoming trial and that the computer would automatically calculate these points, facilitating the subject to focus on the task. Following each trial, audio feedback was provided to indicate correct and incorrect responses. Subjects were remunerated based on the proportion of points earned out of a total of 280 using the following scale: 0–70 points (US $10), 71–140 (US $15), 141–210 (US $20), 211–280 (US $25.00 or gift card). This point-based approach allowed a separation between trial outcomes and dollar amounts, which was reasoned to more adequately adjust for potential differences in the subjective value of dollar amounts across age. This method has been previously used in a behavioral implementation of the current paradigm (Charles F Geier & Luna, 2012). Aspects of this dataset have been published elsewhere (see Paulsen et al., 2015).
2.3 Measures
Trait Sensation Seeking
The self-reported Sensation-Seeking Scale (SSS-V; M. Zuckerman, 1994, 2007), was used to assess trait-like levels of sensation seeking in the current study. The scale consists of 40 items that contain two different descriptive statements (e.g., I like to go off the high board vs. I do not like to go off the high board) and participants are asked to indicate which statement best describes them. This instrument was first administered to participants at the time of their fMRI scan and additionally during two follow-up evaluations spaced approximately 1.5 years apart (M = 1.66 years, SD = .30; M = 1.57 years, SD =. 31). The scale consists of a total sensation seeking score, as well as four 10-item subscales: 1) Thrill and Adventure-Seeking (TAS; “I often wish I could be a mountain climber”), 2) Experience-Seeking (ES; “I like to try new foods I have never tasted before”), 3) Disinhibition (DIS; “I rarely if ever do anything crazy”), 4) Boredom Susceptibility (BS; “I can’t stand watching a movie that I’ve seen before”). Across the three assessment waves, average total scores on this measure were 18.21 (SD = 6.61), 19.25 (SD = 7.11), and 18.76 (SD = 6.79) and reliability ranged from α = .70 −.73.
2.4 Design
The design for this incentivized antisaccade task has been described in detail elsewhere (Paulsen et al., 2015). Subjects were informed that they would see a cue indicating whether correct performance would result in a gain of points (Reward trials), incorrect performance would incur a loss of points (Loss trials), or neither correct nor incorrect performance would affect accrual of points (Neutral trials). Reward and Loss trials were worth +/− 5 points, respectively, which was indicated by the number of filled green or red bars appearing above or below the pre-target central fixation cue. Following each 1.5 second central fixation cue was a 1.5 second preparatory epoch, followed by a 1.5 second saccade event. The display of the saccade event contained a small yellow dot at one of six pseudo randomized peripheral locations; subjects were required to saccade away to the opposite location from the dot upon presentation. Immediately after the saccade, subjects heard a cash register sound for correct responses or a buzzer sound for incorrect responses. Intertrial intervals varied from 1.5–19.5 seconds following an exponential distribution. A total of 56 trials for each Reward, Neutral, and Loss condition were presented across 4 runs. An additional 72 partial trials with either a cue alone or cue and preparatory epoch without a saccade event were also presented to estimate better the hemodynamic response to each event type in other analyses (Ollinger, Corbetta, & Shulman, 2001; Ollinger, Shulman, & Corbetta, 2001). Similar to prior research using this task (e.g., Paulsen, Hallquist, Geier, & Luna, 2014), we collapsed across Cue, Delay, and Response epochs to gain more power in identifying our effects of interest.
2.5 Data Acquisition
Eye-tracking data were collected using a long-range optics eye-tracking system from Applied Science Laboratories (Model 504LRO; Bedford, MA). Eye-position was obtained via pupil-corneal reflection observed in the reflection of a head coil-mounted mirror with 0.5° of visual angle. Video monitoring was also used to ensure compliance. A 9-point calibration was performed prior to the experimental session and between runs when necessary. Stimuli were presented using E-prime software (Psychology Software Tools, Inc., Pittsburgh, PA) and projected onto a flat screen behind the scanner, visible to the subject through the coil-mounted mirror. To provide performance feedback, eye-data were scored on-line using EPrime software. Additionally, eye-data were scored off-line using an in-house automated scoring package developed in R to heuristically match manual scoring. This scoring package has been used in previous analyses (Paulsen et al., 2014).
Correct responses in the antisaccade task were defined as those in which the first eye movement during the saccade epoch with velocity greater than or equal to 30°/s (Gitelman, 2002) was made toward the mirror location of the peripheral cue and extended beyond a 2.5°/visual angle from central fixation. Incorrect responses occurred when the first saccade during the saccade epoch was directed toward the peripheral stimulus and exceeded the 2.5°/visual angle central fixation zone but were subsequently directed to the correct location, indicating that the instructions were being followed. Trials in which no eye movements were generated, or in which the tracker lost fixation, were excluded from analyses, resulting in 14% of trials being excluded.
2.6 MRI Data Acquisition
Imaging data were collected using a 3.0-T Siemens Trio scanner at the Magnetic Resonance Research Center, University of Pittsburgh, Pittsburgh, PA. A single-shot echo-planar imaging sequence sensitive to BOLD contrast (T2*) was performed. The acquisition parameters were TR = 1.5 s; TE = 25 ms; flip angle = 70°; in-plane resolution of 3.125 mm. Twenty-nine 4-mm thick axial slices with no gap were collected, aligned to the anterior and posterior commissure and covering the entire cortex and part of the cerebellum. A 3D volume magnetization prepared rapid acquisition gradient-echo (MP-RAGE) pulse sequence with 192 slices (1-mm slice thickness) was used to acquire structural images in the sagittal plane.
2.7 fMRI data preprocessing
Anatomical scans were registered to the MNI152 template (Fonov, Evans, McKinstry, Almli, & Collins, 2009) using both affine (FSL FLIRT) and nonlinear (FSL FNIRT) transformations. Functional images were preprocessed using tools from NiPy (Millman & Brett, 2007), AFNI (Cox, 1996), and the FMRIB software library (Smith et al., 2004). First, large transient spikes in voxel time series were interpolated downward using the AFNI 3dDespike program. Second, slice timing and motion correction were performed simultaneously using a four-dimensional registration algorithm implemented in NiPy (Roche, 2011). Non-brain voxels were removed from functional images by masking voxels with low intensity and by a brain extraction algorithm implemented in FSL BET. The alignment of subjects’ functional images to their anatomical scan was computed using the white matter segmentation of each image and a boundary-based registration algorithm (Greve & Fischl, 2009). Functional scans were then resampled into 3mm isocubic voxels and warped into MNI152 template space using the concatenation of the functional-structural and structural-MNI152 transforms. Images were spatially smoothed using a 5-mm full-width at half maximum kernel (FSL SUSAN). A .008Hz temporal high-pass filter was applied to remove slow-frequency signal changes.
2.8 First-Level Analyses
Fixed-effects analyses were run using FSL FEAT to generate parameter estimates (PE) at the visit level for each subject for Reward (Rew), Neutral (Neu), and Loss conditions, using the jittered intertrial interval as baseline. Nuisance regressors included the time-courses of two voxels from the right and left lateral ventricles to account for physiological noise, the six motion regressors used in motion correction, and the convolved regressors from error trials, trials that could not be rated due to missing eye-tracking data, or those without a saccade (i.e. partial trials). Thus, only correct trials were used in the analyses, with an event duration of 4.5 s to model the Cue, Preparatory, and Saccade epochs.
2.9 Group-Level Analysis
As the focus of the current study is on reward responsivity, only contrasts with the reward condition of the antisaccade task were examined (i.e., the loss condition was not included in any analysis). The primary contrast of interest was the reward > neutral antisaccade trial (i.e. reactivity to reward stimuli)1. Group analysis of this contrast was performed using a random effects analysis following the FSL FLAME mixed effects approach, which propagates estimation error from single-subject analyses into estimates of group effects (Woolrich, Behrens, Beckmann, Jenkinson, & Smith, 2004). Due to its involvement in various aspects of reward processing and sensation seeking behaviors, our region-of-interest (ROI) analyses were conducted on the bilateral NAcc using an anatomical mask from the Harvard-Oxford sub-cortical structural atlas provided in FSL. Mean BOLD parameter estimates were then extracted for each individual subject data (FSL featquery command) from the right and left NAcc. These values reflect individual differences in the magnitude of neural activation between reward and neutral trials within the NAcc.
In addition to ROI analyses, a voxel-wise exploratory whole-brain (WB) analysis was conducted for the Reward > Neutral contrast. To guard against false positives, a minimum cluster size of 52 contiguous voxels (voxelwise p < .001, cluster threshold of p < .05) was identified for the whole-brain analysis using AFNI’s AlphaSim. Effects reported beyond our a priori ROIs are included only for descriptive purposes.
2.10 Associations with Sensation Seeking
Analyses then examined whether the mean BOLD response to reward in the NAcc was related to trait-levels of sensation seeking, and whether this association varied by age. To assess stable levels of sensation seeking across time, a second-order latent trait model was specified using the 3 repeated assessments of sensation seeking2. In this model, subscales of the SSS-V (i.e., TAS, ES, DIS, BS) were used as indicators of a latent sensation seeking construct at each of the three assessments. These first-order latent sensation seeking factors were then used as indicators of a second-order factor representing time-stable (trait-level) individual differences in sensation seeking across all three time points. After the latent factor model was specified, the average BOLD response to reward, age and their interaction were included as predictors of the higher-order trait sensation seeking construct (for a visual depiction, see Figure 1). All analyses controlled for participant’s gender.
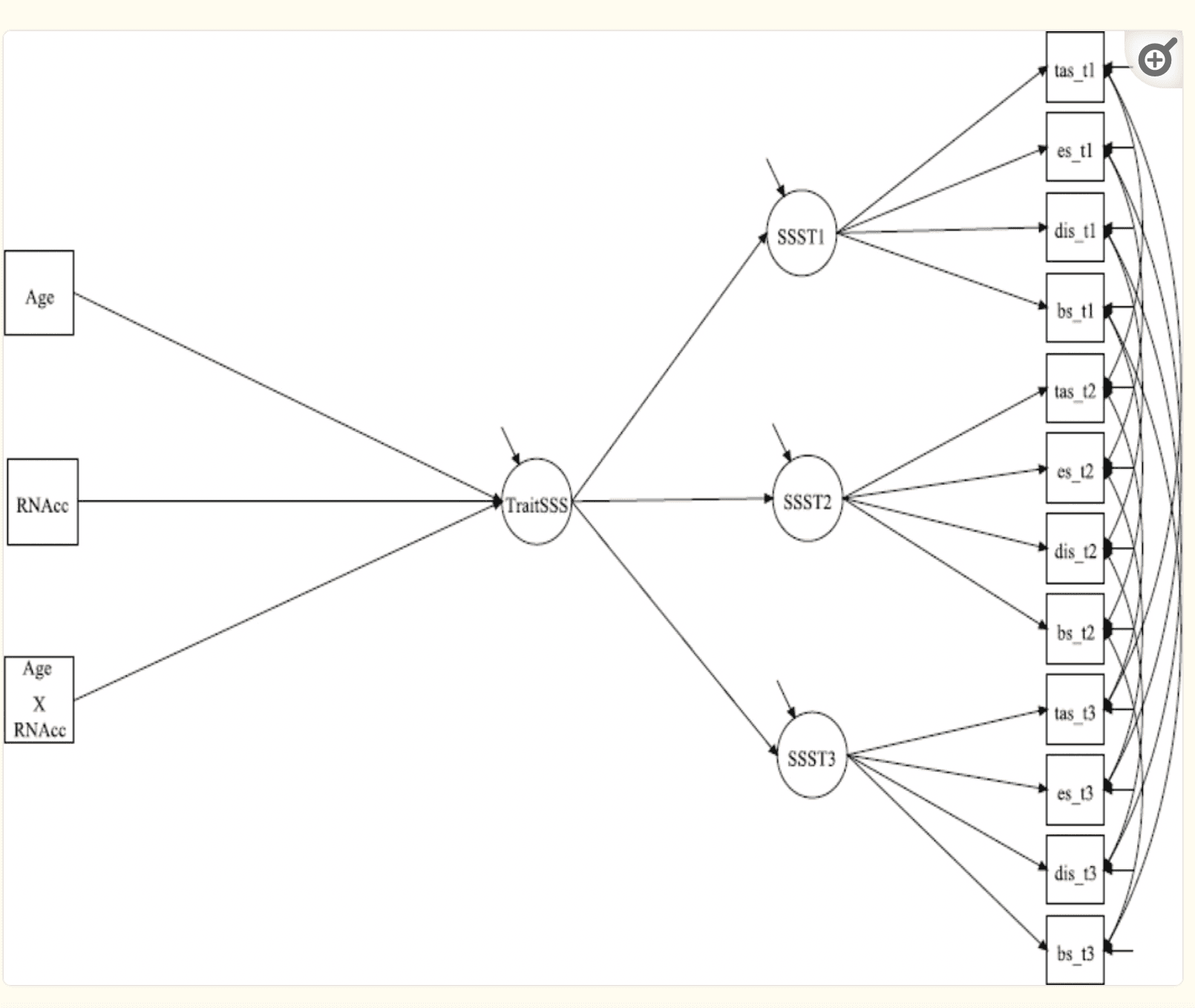
Figure 1 Neural Activation × Age Interaction Predicting Trait
Models were estimated using robust maximum likelihood estimation (MLR) in Mplus 7 (Muthén & Muthén, 1998–2012). Global fit indices used to examine model fit included the comparative fit index (CFI) and the root mean square error of approximation (RMSEA). Cutoff values of .90 or greater were used to indicate acceptable fit and .95 or greater to indicate good fit for CFI (Hu & Bentler, 1999; McDonald & Ho, 2002). RMSEA values between .05 and .10 were considered to represent an acceptable fit, while values less than .05 were considered to indicate good fit (Browne & Cudeck, 1993; McDonald & Ho, 2002).
In addition, longitudinal invariance testing was conducted to determine if the sensation seeking construct was being measured in a consistent manner across the study window. First, a baseline configural model was specified, allowing factor loadings and intercepts to vary across time. Subsequent models were specified by adding parameter constraints, until arriving at a final invariance model with factor loadings, intercepts, factor covariances, and item residual variances constrained to equality across each assessment wave. These models were compared via (Satorra-Bentler) chi-square difference testing, wherein a non-significant chi-square indicates that the more constrained model should be adopted. However, as the chi-square difference test is sensitive to sample size and violations of the normality assumption, we also assessed change in CFI and RMSEA fit indices across models. Changes in CFI equal to or less than .01 and changes in RMSEA of equal to or less than .015 have been proposed as demonstrating evidence of model invariance (Chen, 2007; Cheung & Rensvold, 2002).
3. Results
3.1 Behavioral
Study participants’ overall correct response rate (‘accuracy’) was high for the Reward (M = .92, SD = .08) and Neutral (M = .89, SD = .11) conditions, however, accuracy was significantly greater for Rewarded trials (t(135) = 4.09, p < .001). Similar to previously reported findings in research that has spanned a large developmental window (e.g., B. Luna, Garver, Urban, Lazar, & Sweeney, 2004; Paulsen et al., 2014), accuracy rates also improved with age and followed an inverse trajectory for both Reward (B = .45, t(1, 138) = 5.83, p < .001; R2 = .20) and Neutral (B = .42, t(1, 138) = 5.39, p < .001; R2 = .18) trials (see Figure 2). There was no evidence of an association between accuracy rates and sensation seeking for the reward (r = .12, p = .18) or neutral condition (r = .07, p = .40), or any evidence that age moderated this association.
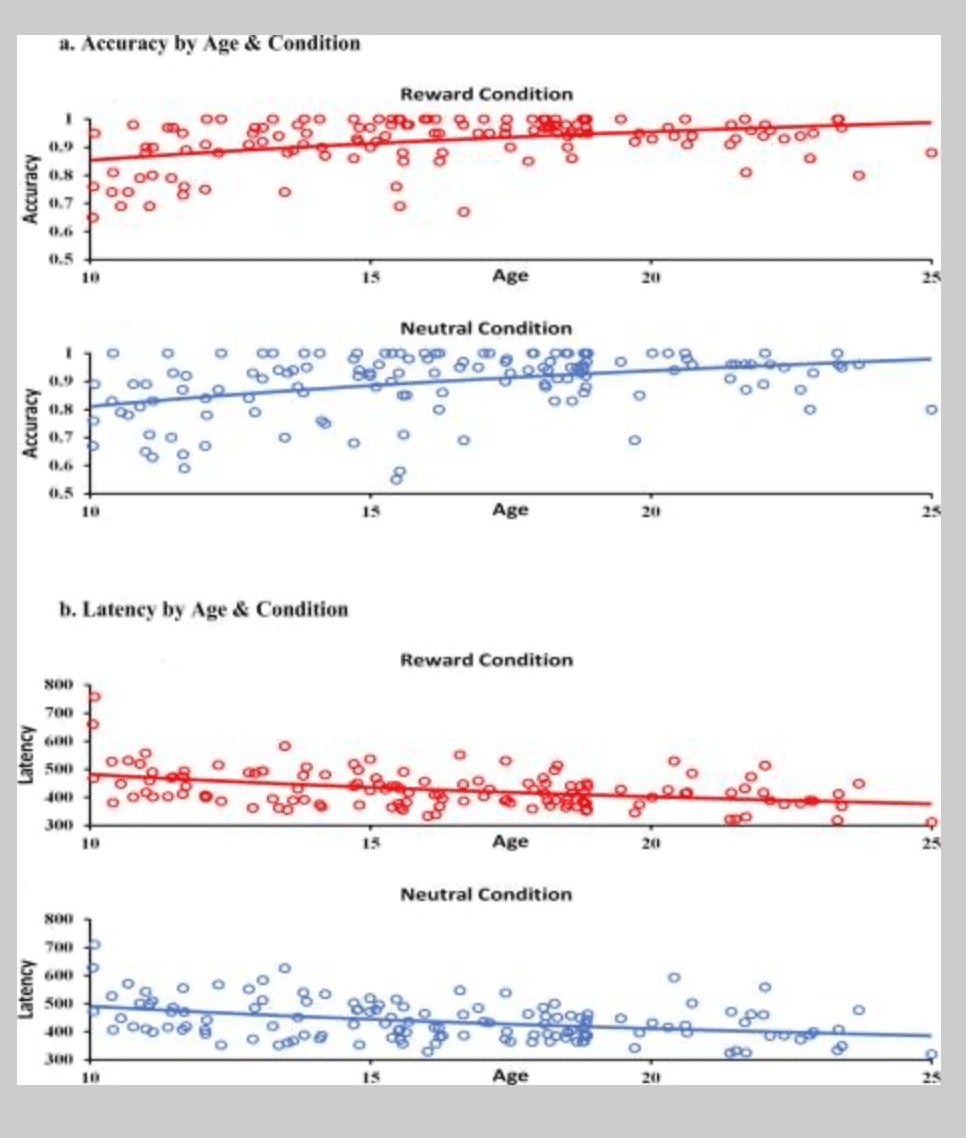
Figure 2 Antisaccade Performance during Reward and Neutral Trials
Saccade latencies for correct responses were also examined to assess participants’ reaction time. Similar to the accuracy rate findings, a significant difference was found between Reward and Neutral conditions. Reaction times during Rewarded trials (M = 427.86, SD = 66.34) was significantly less than during Neutral trials (M = 436.76., SD = 70.41; t(135) = −4.21, p < .001). In addition, these results also indicated that an inverse trajectory provided an appropriate fit to the data, whereby reaction times decreased across age for the Reward (B = −.43, t(1, 138) = −5.44, p < .001; R2 = .18) and Neutral (B = −.40, t(1, 138) = −5.02, p < .001; R2 = .16) conditions (see Figure 2). Finally, as with accuracy rates, there was no evidence of any relationship between latency and sensation seeking in the reward (r = −.08, p = .38) or neutral conditions (r = −.05, p = .58).
3.2 Latent Trait Modeling of Sensation Seeking
Prior to investigating associations between neural activation and participant’s levels of sensation seeking, longitudinal invariance of the sensation seeking construct was examined. The baseline configural model was found to provide a good fit to the data (χ2 = 45.64, df = 39, p = .215; CFI = .987, TLI = .979, RMSEA = .035). Similarly, the more constrained, final invariance model was also found to provide good model fit (χ2 = 72.91, df = 61, p > .05; CFI = .977, TLI = .976, RMSEA = .037; also see Table S1). Comparing these two models revealed negligible differences among the absolute fit indices (i.e., CFI, TLI), while results of chi-square difference testing also indicated there was no significant difference between these models (χ2 = 27.31, df = 22, p > .05). Therefore, these results were considered to support the longitudinal invariance of the sensation seeking construct in the current study.
The higher-order latent trait model of sensation seeking fit the data well (χ² (54) = 66.30, p = .121, RMSEA = .040, CFI = .977; see Figure 1). Analyses were also conducted to compare the fit of this latent trait model to that of a higher-order latent growth curve model. A comparison of these nested models provided evidence that the latent trait model fit the data better (BIC = 4615) compared to the growth curve model (BIC = 4924). Subsequent to these model testing steps, we investigated whether there was an association between brain activation to reward and trait-like levels of sensation seeking, as well as if an age effect moderated this relationship.
3.3 Functional Imaging Results
Mean ROI activation from the reward > neutral contrast was extracted for the right (MNI coordinates: 40, 72, 33) and left (MNI coordinates: 48, 67, 36) NAcc. There was no evidence of a linear or (inverse) quadratic association between participant’s age and reward activation in either the right (linear- β = .05, p = .53; inverse- β = −.02, p = .79) or left (linear- β = .09, p = .25; inverse- β = −.05, p = .54) NAcc regions. This is similar to our previous study using overlapping data (Paulsen et al., 2015), where we found that NAcc engagement during reward was robust across ages undermining the ability to uncover age effects.
In addition, results indicated there was a main effect of age on trait sensation seeking, but no main effect of NAcc neural activation predicting this outcome (see Table 1 and Table S2)3. However, further analyses revealed a significant age-by-activation interaction in the prediction of sensation seeking for the right NAcc (b = .14, SE = .09, β = .20, p < .05)4. An examination of Akaike (AIC) and Bayesian (BIC) Information Criterion also demonstrated improved model fit when this interaction term was included in the model (AIC = 4925; BIC = 4915) compared to a model in which it was not included (AIC = 4927; BIC =4918). At earlier ages, higher levels of reward-related activation in the right NAcc were associated with lower sensation seeking. However, at later periods of development an opposite pattern emerged. That is, reactivity to reward was associated with higher sensation seeking during later periods of development (see interaction plot in Figure 4). The overall R2 for this model without the interaction term included was .16, while inclusion of the interaction term increased the value of R2 to .21 (ΔR2 = .05). This interaction was not found for the left NAcc (β = .15, p = .07). To place these findings into a more developmentally informative context, we further probed this interaction effect using the PROCESS macro (Hayes, 2015) to employ the Johnson-Neyman statistical approach (Johnson & Fay, 1950; Potthoff, 1964). This technique identifies ranges of a moderator variable (e.g., age) for which the influence of the predictor (e.g., NAcc activation to reward) on the outcome is statistically significant. This analysis indicated that prior to 12 years of age, increased NAcc reactivity was significantly associated with lower levels of sensation seeking, whereas at ages greater than 17, NAcc activation was significantly associated with increased sensation seeking (see Figure 4).
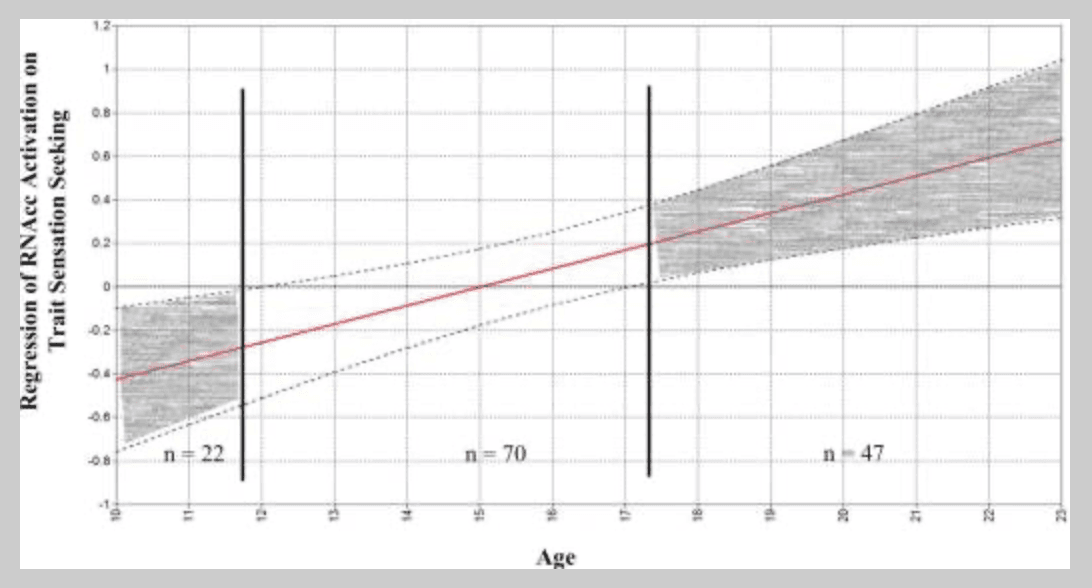
Figure 4 Regions of Significance PlotNote. Johnson-Neyman regions of significance plot. The figure shows the simple slope on the y-axis for the relationship between RNAcc activation and Trait Sensation Seeking, plotted against participant’s age on the x-axis. The dashed lines represent the upper and lower limit of the 95% confidence intervals surrounding the simple slopes (solid line) at each level of the moderator (age). The vertical solid lines represent the value of age at which the lower and upper limits of the confidence interval crosses the zero point and the relationship between RNAcc activation and Trait Sensation Seeking becomes significant. n’s = the number of participants within each of the age bins from the region of significance plot.
Table 1
Main Effects and Interactions of Neural Activity and Age Predicting Sensation Seeking
Trait Sensation Seeking | TAS Subscale | ES Subscale | DIS Subscale | BD Subscale | |||||||||||
B | SE | P | B | SE | P | B | SE | P | B | SE | P | B | SE | P | |
Main Effects | |||||||||||||||
Age at T1 | .22 | .10 | .03 | .20 | .11 | .08 | .50 | .09 | <.001 | .13 | .10 | .20 | .11 | .09 | .23 |
RNAcc | .05 | .09 | .48 | .12 | .10 | .24 | .00 | .11 | .97 | .08 | .09 | .37 | −.04 | .10 | .65 |
LNAcc | .10 | .08 | .23 | .06 | .10 | .53 | .04 | .11 | .71 | .10 | .08 | .22 | .09 | .09 | .31 |
Interactions | |||||||||||||||
Age X RNAcc | .20 | .09 | .03 | .01 | .12 | .94 | .18 | .09 | .05 | .35 | .15 | .02 | .09 | .09 | .33 |
Open in a separate window Notes: Above analyses control for participant genderTAS = Thrill and Adventure-Seeking Subscale; ES = Experience-Seeking Subscale; DIS = Disinhibition Subscale; BD = Boredom Subscale
3.4 Exploratory Analyses
Exploratory whole-brain analyses also supported the NAcc findings from our ROI analysis. A significant cluster of neural activity (79 voxels) in the right NAcc and overlapping our ROI analysis exceeded whole-brain cluster correction for the Reward > Neutral condition (peak activation: 40, 66, 38, MNI coordinates; see Figure 3). As with the ROI results, an age-by-activation interaction (using the mean parameter estimate of this cluster) was found to be a significant predictor of trait levels of sensation seeking (b = .20, SE = .07, β = .25, p = .003). The whole-brain analysis also identified a small number of other regions outside of our hypothesized ROIs, that elicited significant activation in the Reward > Neutral condition. However, none of these regions demonstrated a significant main or interaction effect with participant’s age or level of sensation seeking. A complete table of results from the whole-brain analysis is available in the supplemental online materials (Table S3).Figure 3Whole Brain Activation in Reward > Neutral Contrast Notes. BOLD activation obtained from whole-brain analysis for clusters exhibiting significantly greater activity in the reward compared to neutral condition (minimum cluster size = 52 contiguous voxels; voxel-wise p < .001; cluster threshold of p < .05). Table S1 provides cluster size as well as coordinates and z-statistic for the peak voxel of each significant cluster
4. Discussion
This study characterized unique developmental effects of the relationship between sensitivity to reward and trait-levels of sensation seeking. We found that during earlier periods of development (i.e., late childhood to early adolescence), increased NAcc response to reward was linked to lower levels of sensation seeking at follow-up times. However, the period of late adolescence to early adulthood marked a transition point whereby increased reward activation was associated with higher levels of sensation seeking. This is the first empirical evidence, of which we are aware, to suggest that reward sensitivity may serve as an important biomarker that confers unique vulnerabilities for engaging in high-risk behaviors differentially across development.
The finding that NAcc reactivity exhibited differential associations with sensation seeking across development lends support to prior research suggesting an association between developmental changes in reward responsivity and cognitive control (Paulsen et al., 2014). Several studies now show that although overall NAcc response to reward cues may be similar across development, this responsivity is only associated with risk-taking behaviors in late adolescence and early adulthood, but not during childhood (Galvan et al., 2007; Luking, Luby, & Barch, 2014). This suggests that reward reactivity in the NAcc may reflect different aspects of development during earlier compared to later developmental stages. While research such as the dual systems model suggests that reward sensitivity follows a curvilinear pattern across adolescence, findings from this study indicate that important functional change may occur even if reward reactivity remains robust across development. It is also possible that changes in the relationship between NAcc reactivity and sensation seeking across development are due, in part, to changes in the functional role of DA during this time. Findings from a large body of literature suggest that there is substantial change in the DA system during adolescence, including shifts in firing rate patterns and overall levels of DA (e.g., Galvan, 2010; McCutcheon & Marinelli, 2009; Padmanabhan et al., 2011; Wahlstrom et al., 2010). It may be that these changes impact the general role of DA and/or influence the way in which neural regions within the DA system interact, which in turn affects behavioral responses.
It is also worth noting that the interaction effect found in this study appears to be driven largely by the disinhibition (DIS) and experience-seeking (ES) subscales of the sensation seeking measure. Compared to aspects of restlessness and socially acceptable means of arousal seeking (e.g., playing sports) assessed by the boredom (BS) and thrill and adventure seeking (TAS) subscales of the measure, items on the DIS and ES scales appear to tap into more atypical, high-risk behaviors such as defying authority and use of illicit substances (M. Zuckerman, 1994; M. Zuckerman & Como, 1983). However, future research that parses out these associations in a more fine-grained manner is needed before arriving at a clearer conclusion.
Together, these results provide evidence that the relationship between striatal indices of reward processing and sensation seeking increasingly couple at older adolescent ages when sensation seeking typically peaks. Earlier in development, indices of striatal sensitivity to reward processing may be a marker of developmental timing where more advanced maturation is coupled with less sensation seeking.
4.1 Limitations
This study was characterized by a number of strengths including the use of a relatively large sample, repeated assessments of study outcomes, and the incorporation of neuroimaging data into an SEM framework to assess prospective prediction. However, several limitations should also be noted. First, this study focused on a convenience sample of healthy participants and may not generalize to at-risk populations. Non-generalizable samples have the potential to distort aspects of brain-behavior relationships (Falk et al., 2013) and youth in the current study may not be representative of those most likely to engage in high risk behaviors. Some research suggests this is particularly important to keep in mind when interpreting findings related to clinically significant adolescent risky behavior that are based on brain responsivity of neurotypical subjects (for more detailed consideration of this topic, see Bjork & Pardini, 2015). In addition, the current investigation did not examine the relationship between sensation seeking and reward reactivity across distinct task specific phases of reward processing (e.g., anticipation and receipt of rewards). Although findings across several fMRI (for a review, see Plichta & Scheres, 2014), and PET studies (Leyton et al., 2002; Lind et al., 2005) have revealed no general differences in this pattern of associations (but also see Richards, Plate, & Ernst, 2013), future research specifically focused on disaggregating and testing these effects is needed.
The latent trait model of sensation seeking included data collected during the T1 assessment, at which time study fMRI data was also collected. Thus, the predictive SEM model was not fully prospective in its design and results should be interpreted with this in mind. Potential aspects of dynamic change that may stem from time-specific variations in the sensation seeking construct were not examined in this study. In addition, data from the current study was collected using an age-accelerated approach and developmental effects were assessed, in part, via the use of age data collected cross-sectionally. To help ensure that any mean shifts in sensation seeking were controlled for, sensation seeking scores from each assessment wave were regressed onto participant’s age at each phase (i.e., T1, T2, and T3). However, we note that future investigations should examine study findings in the context of a more traditionally implemented longitudinal design, consisting of a larger number of follow-up assessments. Such a design may facilitate easier implementation of methods to provide a more direct assessment of issues related to developmental change in sensation seeking, and for modeling the joint development of brain activation and sensation seeking (e.g., bivariate latent growth curves; growth mixture modeling). Finally, the current study focused on age as a moderating influence between reward response and sensation seeking. However, future research may also wish to consider other potentially viable models, such as neural response to reward mediating the path from age to sensation seeking, or other more complex relations (e.g., moderated mediation/mediated moderation models).
4.2 Conclusions
Although sensation seeking may serve an adaptive role in some contexts, it has also been linked to an individual’s propensity to engage in numerous high risk behaviors, many of which have injurious and costly outcomes. Understanding the mechanisms that underlie sensation seeking is essential for early identification and prevention purposes. Here we show that sensation seeking appears to demonstrate a unique association with reward-related activity in the nucleus accumbens across development. Developmental findings indicate that this response during late adolescence and early adulthood may provide important insight into sensation seeking and risk taking behaviors that persist into later development. Although these findings must be replicated in other samples, this suggests that reward sensitivity exhibited in the nucleus accumbens region may serve as a marker to the propensity for sensation seeking that may inform models of atypical development including substance use disorders and delinquent behaviors.