Abstract
The Research Domain Criteria (RDoC) initiative provides a strategy for classifying psychopathology based on behavioral dimensions and neurobiological measures. Neurodevelopment is an orthogonal dimension in the current RDoC framework; however, it has not yet been fully incorporated into the RDoC approach. A combination of both a neurodevelopmental and RDoC approach offers a multidimensional perspective for understanding the emergence of psychopathology during development. Environmental influence (e.g., stress) has a profound impact on the risk for development of psychiatric illnesses. It has been shown that chronic stress interacts with the developing brain, producing significant changes in neural circuits that eventually increase the susceptibility for development of psychiatric disorders. This review highlights effects of chronic stress on the adolescent brain, as adolescence is a period characterized by a combination of significant brain alterations, high levels of stress, and emergence of psychopathology. The literature synthesized in this review suggests that chronic stress-induced changes in neurobiology and behavioral constructs underlie the shared vulnerability across a number of disorders in adolescence. The review particularly focuses on depression and substance use disorders; however, a similar argument can also be made for other psychopathologies, including anxiety disorders. The summarized findings underscore the need for a framework to integrate neurobiological findings from disparate psychiatric disorders and to target transdiagnostic mechanisms across disorders.
Introduction
Stress has significant negative effects on the physical and mental health of Americans, irrespective of gender, race, and age.1 Stress occurs when mental, emotional, and/or physical demands increase beyond the regulatory capacity of an organism,2 and the impact on an organism may differ depending on the frequency, magnitude, and duration of the stress. While moderate levels of stress can be adaptive, stress persisting for long periods can have negative consequences on the well-being of the organism.3 Several preclinical and clinical studies have shown that chronic stress produces alterations in gray and white matter of the brain, affecting healthy neural communication through changes in brain circuits.4–6 Furthermore, chronic stress has been associated with several psychiatric illnesses, such as depression, anxiety, post-traumatic stress disorder (PTSD), substance use disorder (SUD), and personality disorders.4,7,8 The impact of chronic stress on psychopathology is so important that it is included as a construct in the Research Domain Criteria (RDoC) matrix, an initiative introduced by the National Institute of Mental Health (NIMH) to bridge the gap between basic neuroscience and psychiatry.9
In this review, we provide an RDoC perspective on the neurobiological consequences of chronic stress in adolescence with an overall objective of identifying neurobiological and behavioral commonalities that cross diagnostic categories. A bottom-up approach is taken starting with neural circuits as they relate to behavioral syndromes. First, we describe the effects of chronic stress on neurotransmission, neural substrates, and circuits. Second, effects of stress on behavioral constructs, specifically anhedonia, are summarized. We note that anhedonia is a phenotype that is observed in several psychiatric illnesses10 and is a widespread phenomenon in adolescence.11 We subsequently provide evidence suggesting that the same corticolimbic circuits modified by stress may underlie stress-induced anhedonia, thus providing a link between biology and behavior. We include studies that examine effects of diverse temporal patterns of the stressor, which will help elucidate the precise nature of behavioral and neurobiological changes and whether the timing of the stressor produces differential outcomes (Figure 1). Finally, we propose a heuristic model demonstrating chronic stress-induced abnormalities in circuits and behaviors that are shared across psychiatric disorders in adolescence. We specifically focus on depression and SUD, given the extensive comorbidity and similar underlying neurobiological mechanisms.12,13
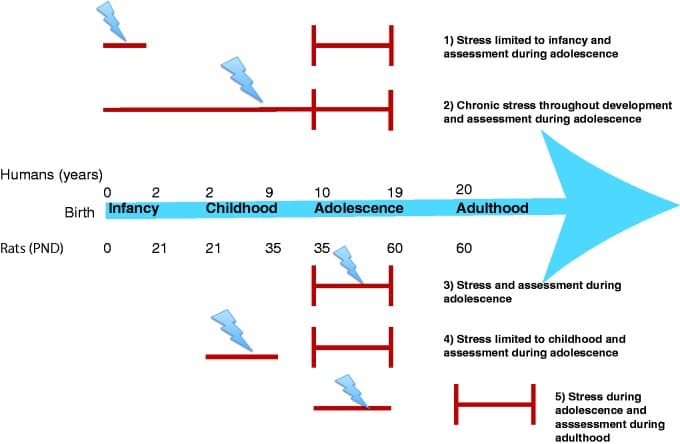
Figure 1. Timeline of developmental stages in humans and rodents and diverse temporal patterns of chronic stress. Lightning bolt indicates timing of stress and red vertical lines indicate timing of assessment. Description for each row is provided on the right.
RDoC: Sustained Threat Construct
The Diagnostic and Statistical Manual of Mental Disorders (DSM) is a system designed to provide a clinical framework for signs and symptoms of psychiatric diagnoses. While development and use of the DSM has improved the reliability of clinical diagnosis, it is not without limitations.14,15 One of the critical challenges facing diagnosis of psychiatric disorders is the lack of cohesion between presenting symptoms and the underlying pathophysiology and dysfunction in brain circuits. The absence of identified neural mechanisms may impact the development of novel therapeutic agents in psychiatry, as neural mechanisms underlying symptoms are not well characterized and often overlap across diagnostic categories. To address this problem, the RDoC initiative by NIMH was introduced to help identify fundamental aspects of behaviors and circuits that may span multiple disorders with the ultimate goal of providing focal treatment targets for psychiatric patient populations.16
The RDoC matrix includes five domains reflecting major systems of cognition, emotion, motivation, and social behavior: negative valence systems; positive valence systems; cognitive systems; social processing; and arousal and regulatory systems. Each domain is further divided into lower level constructs representing specific dimensions of behaviors. Units of analysis include genes, molecules, cells, circuits, physiology, behaviors, self-reports, and paradigms. The current review focuses on analyzing circuits and behaviors utilizing the sustained threat construct (representative of chronic stress). NIMH defines the sustained threat construct as “an aversive emotional state caused by prolonged exposure to internal and/or external condition(s), state(s), or stimuli that are adaptive to escape or avoid.” Furthermore, the review will consider developmental trajectories across adolescence as a critical element of the RDoC matrix, which may enhance the neurobiological-based understanding of psychiatric illnesses as evolving neurodevelopmental patterns.17,18
Chronic Stress and Adolescence: Key Definitions
Adolescence is defined as the period between childhood and adulthood, beginning with the onset of puberty and characterized by changes in hormonal levels and consequent physical, psychological, and social changes. Adolescence roughly corresponds to the period between 10 and 19 years,19though there is evidence that adolescence may extend up to 25 years of age.20 In rodents, this developmental stage is defined as the period between 35 and 60 days of age.21 In humans, adolescence is accompanied by increased exposure to stressors22,23 as well as behavioral changes and emergence of psychiatric illnesses.24,25 Further, there is a strong association between chronic stress and psychopathology in adolescence, with stress linked to depression, anxiety, and other internalizing and externalizing problems.26–28 For the purpose of clarity, this review will focus solely on chronic stress represented by the “sustained threat” construct in the RDoC matrix. As mentioned above, this is defined as “environmental events or chronic conditions that objectively threaten the physical and/or psychological well-being of an individual.”26 There are number of sources of chronic stress in adolescence. These include but are not limited to poverty and economic hardship,29 personal or parental chronic illness,30,31 and chronic maltreatment, abuse, and neglect.32
Vulnerability During Adolescence
Using a wide range of methodologies, investigators have reported that adolescence is a vulnerable period for stress, predisposing adolescents to stress-related psychopathologies. This section summarizes neuroanatomical and neuroendocrine evidence from animal and human studies, which may account for susceptibility to stress-induced neural dysfunction during adolescence.
Dynamic changes occur in the brain throughout the course of development. There is an overproduction of synapses early in development followed by substantial synaptic loss and pruning.33 Due to the extensive synaptic and cellular remodeling, adolescence represents a time of developmental neuroplasticity, wherein circuits are sculpted by the environment and are malleable to experience, aiding the transition to adulthood.34–36 Adolescence also has been associated with diminished plasticity in circuits underlying behavioral domains that show deficits in adolescence (e.g., vmPFC in fear extinction), suggesting that the direction of change in plasticity may be region-specific.37 Since fear extinction is important for recovery from stress, these results highlight delayed recovery from stress exposure in adolescents.
Further, structural and functional reorganization in limbic and cortical structures occurs during adolescence.38–43 Animal and human studies have demonstrated increases in amygdala and hippocampal volumes in the early stages of puberty.44–50 The region that is most dynamic during adolescence is the prefrontal cortex (PFC), which increases in volume from childhood to early puberty and undergoes thinning and synaptic pruning throughout adolescence and young adulthood.38,41,43 Based on rodent models51,52 and human imaging studies,53–55 a number of researchers56–60 have proposed a neurobiological model characterized by an imbalance between the bottom-up limbic and prefrontal top-down circuits during adolescence. According to this model, the limbic regions functionally mature earlier than cortical regions during adolescence, as opposed to childhood where both systems are developing and adulthood where both systems are mature.56,61,62 The discordant development of limbic and cortical systems may explain some of the psychological and behavioral changes seen during adolescence, including emotion dysregulation, risk-taking, and increased sensitivity to rewards.63–66Together, these studies suggest that adolescence is a sensitive period characterized by dynamic changes, wherein environmental factors such as chronic stress can alter neural systems,67 especially those that are still developing.68,69
In addition to the neuroanatomical changes mentioned above, there are significant neuroendocrine changes that occur during this developmental period.70 The hypothalamic-pituitary-adrenal (HPA) axis is a major neuroendocrine system that is activated in response to stress and has been shown to initiate the stress response. In response to stress, the corticotropin-releasing factor (CRF) is produced in the paraventricular nucleus of the hypothalamus (PVN).71 CRF then acts in the anterior pituitary to release the adrenocorticotropic hormone (ACTH), which is released into circulation.72ACTH acts on the adrenal cortex, where it induces the secretion of glucocorticoids. Glucocorticoids are the effectors of the HPA axis and produce physiological changes in response to stress.73 While the acute stress response is adaptive, prolonged stress can cause dysregulation of the HPA axis leading to abnormal glucocorticoid levels.74 Importantly, glucocorticoids can cross the blood–brain barrier, act on receptors, and regulate genes involved in neurogenesis, neuroplasticity, and neurotransmission in the PFC, hippocampus, and amygdala.75–79Furthermore, the PFC has higher levels of glucocorticoid receptor mRNA in adolescence than at any other period in development, suggesting that the PFC may be sensitive to glucocorticoid regulation80 and hence susceptible to stress-induced changes in adolescence.81 Concordantly, increased glucocorticoid levels in response to early stress modulate the connectivity between the PFC and amygdala. This altered connectivity is associated with enhanced vulnerability to internalizing disorders in adolescence, suggesting that the association between stress and psychopathology may be mediated in part by the interaction of the HPA axis with developing brain circuits.82Interestingly, animal studies of the HPA axis have shown that the stress response is significantly different in adolescent compared to adult rats,6 with adolescent rats demonstrating prolonged release of glucocorticoids in response to several stressors.83–86 Further, adolescent rats showed potentiated release of stress hormones in response to repeated stress in contrast to adult rats, which showed a habituated stress response.87 Chronic-foot-shock stress in adolescence caused HPA axis dysregulation in adulthood, which may underlie the associated abnormal behaviors.88 Human adolescents also have demonstrated increased basal and stress-induced HPA activity.89 The above evidence suggests that elevated stress-induced HPA axis reactivity in adolescents may affect the architecture of the brain. Thus, the continued maturation of stress-responsive brain regions and increased stress-hormonal responses may serve as converging factors in making adolescence a particularly vulnerable period for stress-induced neural dysfunction and psychopathology.
In the following sections, we discuss the effects of chronic stress on different brain structures during adolescence. We specifically focus on the PFC, amygdala, and hippocampus for the following reasons: (1) these regions show significant modifications in response to stress in both animal and human models due to high glucocorticoid receptor expression (see below); (2) the top-down PFC control on subcortical limbic structures like the amygdala and hippocampus undergoes changes during adolescence and underlies characteristic adolescent behaviors; and (3) the concerted activity of the PFC, amygdala, and hippocampus subserves reward and limbic-related functions, including reward sensitivity and fear regulation.90–94
Effects of Chronic Stress on the PFC
The PFC often is considered a single brain region; however, it is composed of subregions that differ in cytoarchitecture, connectivity, and functional properties. A number of excellent reviews on this topic are available.95,96The dorsolateral (dl) and ventrolateral (vl) PFC are considered executive regions of the PFC, with the dlPFC involved in working memory, response selection, cognitive flexibility, and abstract reasoning.97–99 The vlPFC plays a role in stimulus selection, task-switching, reversal learning, and short- and long-term memory.100–102 The ventromedial (vm) PFC is a critical node in the reward system consistently linked to reward outcome and subjective pleasure.103,104 Relevant to the focus on the sustained threat construct, several studies have demonstrated a prominent role for the vmPFC in fear extinction.105,106 Further, the vmPFC is important for emotion regulation,107decision-making, and social function,108,109 showing considerable overlap in function with the orbitofrontal cortex (OFC). The OFC is one of the last regions to fully develop in the human brain and has been implicated in inhibition and self-regulation of social-emotional behavior.110,111 Although the anterior cingulate cortex (ACC) is not traditionally considered to be part of the PFC, this region is included in the review given its connections to the PFC and limbic regions and its role in cognitive control of emotion regulation.112 Despite the described regional specialization, there are shared functions among PFC areas. Broadly, the dlPFC and vlPFC are important for executive functioning while the OFC, vmPFC, and ACC are important for reward and emotion regulation. Figure 2 demonstrates PFC subregions and connectivity with the subcortical limbic regions.
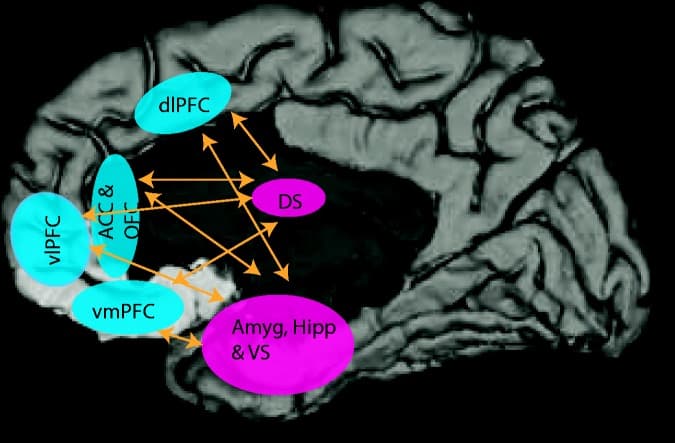
Figure 2. Corticolimbic circuits critical for executive function, reward processing, and emotion regulation.
Rodent studies have demonstrated that chronic restraint stress restricted to the adolescent period induced dendritic atrophy in PFC neurons in adolescent males and females.113 Moreover, markers of cortical synaptic plasticity have been significantly reduced following social isolation in adolescence.114,115 After the cessation of isolation stress, the changes in synaptic plasticity persisted for longer in adolescents compared to adults.116Together, these studies suggest that chronic stress causes structural changes in the adolescent rodent PFC.
Human studies investigating the effects of chronic stress on the PFC during this developmental period have reported similar results. For example, a diffusion-tensor imaging (DTI) study in previously institutionalized participants (average institutionalization time = 29.2 months) showed white matter abnormalities in the PFC, as well as deficits in white matter tracts connecting the temporal cortex and PFC, during adolescence.117 Higher cumulative life stress, maltreatment, and physical abuse in adolescents all have been associated with smaller PFC volumes, specifically the vmPFC, ACC, and OFC regions.111,118,119 Physically abused adolescents (mean age = 12) showed reduced OFC volumes as compared to controls,111 although the duration of the physical abuse in this study was unclear. Further, portions of the PFC were smaller in adolescents who reported higher cumulative stress during their lifetime, which was associated with poor executive functioning.118 Together, these studies suggest that chronic stress throughout development111,118 as well as stress restricted to early postnatal years117 may significantly alter PFC structure and volume measured during adolescence. The structural modifications of the PFC may have functional implications such as reduced top-down control of emotion regulation.
Effects of Chronic Stress on the Amygdala and Hippocampus
The amygdala and hippocampus are important nodes in the limbic system and are critical for processing emotion, motivation, and memory. The amygdala plays an important role in identifying emotional significance120 and is involved in associative learning, fear conditioning, and generating behavioral responses.121,122 The hippocampus has been associated with learning, declarative and spatial memory functions123,124 as well as mood regulation.125 Furthermore, both these structures play pivotal roles in coordinating behavioral, emotional, and endocrine responses to stress.126,127 Animal studies investigating the effects of chronic stress on the amygdala and hippocampus during adolescence have consistently observed significant effects. For example, chronic restraint stress during early puberty has been shown to increase dendritic length but decrease spine density in the basolateral nucleus of the amygdala (BLA) in rats, which correlated with stress-induced changes in fear generalization.128 Social isolation in adolescent rats increased intrinsic excitability of BLA pyramidal neurons, which may be the neurobiological mechanism underlying increased anxiety during adulthood.129 Further, postnatal stress caused persistent elevation of c-Fos in the BLA and deficits in fear regulation across development such that mice exposed to stress restricted to the preweaning period were unable to suppress fear responses when assessed during adolescence and early adulthood.130 Together, these studies reinforce the notion that exposure to stress during infancy130 and adolescence128,129 alters amygdala structure and function, the effects of which are evident during adolescence as well as later in life.
The hippocampus also has been shown to play a salient role in response to stress. In animal studies, morphological investigations showed reductions in dendritic branching in the hippocampus of adolescent male and female rats after prolonged restraint stress during adolescence.113 Further, chronic variable physical stress during the peripubertal-juvenile period in rats slowed the increase in hippocampal volume from late adolescence to early adulthood.49 Together, these studies suggest that chronic stress during adolescence may profoundly affect the hippocampus during the same developmental period. Adolescent stress also may produce changes that are evident during adulthood. For example, a history of chronic restraint and social instability stress during adolescence decreased neurogenesis in adult female rats but increased neurogenesis in adult male rats.131–133 A recent study demonstrated that stress during infancy caused accelerated hippocampal behavioral development as well as rapid decline of neuronal proliferation and differentiation markers in the hippocampus in adolescence, indicative of precocious maturation.134 This study indicates that chronic stress during postnatal life may cause an accelerated trajectory of hippocampal development in adolescence. Although a causal link has not been established, these stress-induced changes in hippocampal structure and function may be related to the learning and memory deficits observed in adulthood.132,133 Some caution is warranted in translating adolescent rat studies to adolescent humans especially involving the hippocampus since the developmental trajectory of the hippocampus in the two species is somewhat different.5 For example, a longitudinal study in humans showed that total hippocampal volume from age 4 to 25 measured by magnetic resonance imaging (MRI) remained unchanged bilaterally,40,135 while in a rodent study, immunohistochemical analysis showed that neurogenesis in the hippocampus continued into adulthood.136
Although rodent studies have consistently shown alterations in amygdala and hippocampus following stress in adolescence, human imaging studies have been contradictory with meta-analyses revealing inconsistent findings.137,138 A morphometric study in 10-year-old children exposed to continuous poor maternal care, related to maternal depressive symptomatology showed an increase in amygdala volume compared to children who were not exposed to this stress.139 In agreement, previously institutionalized children who were adopted (average age of adoption = 24 months) had higher amygdala volumes compared to controls, when scanned during childhood to early adolescence.140 Interestingly, the length of stay in the orphanage was positively associated with amygdala volume, suggesting that the duration of the stress may have significant bearing on the severity of the outcome. In addition to these structural findings, a functional MRI (fMRI) study in preadolescents showed that adverse rearing conditions during the postnatal period was associated with atypically high amygdala activation in response to fearful faces and distracter stimuli.130,141 Thus, stress during infancy has been shown to modify amygdalar structure and function when evaluated several years after the stressor. Further, stressors experienced later in development can also significantly impact the amygdala. For example, in a study of healthy adolescents, the total number of stressors after the age of 4 was positively correlated with amygdalar activation in response to emotional faces.142 Moreover, in a study of male adolescents, lower perceived socioeconomic status (SES) during childhood and adolescence predicted higher amygdala activation to fearful faces.143 Thus, stress exposure in childhood and adolescence may also shape amygdala function. These studies were in contrast to morphometric studies reporting smaller amygdala volumes144,145 or no differences.146,147 in individuals who had suffered childhood maltreatment and were scanned during adolescence. Differences in duration and the timing of the stressor may have contributed to these divergent findings underscoring the need for documenting the precise details of stress exposure in human studies for meaningful interpretation of neurobiological findings.
Neuroimaging studies with young adolescents from low SES households and those with a history of physical abuse showed lower hippocampal volume than non-abused controls.145,148 On the other hand, children and adolescents with maltreatment-related PTSD showed no change in hippocampal volumes as compared to healthy controls.149,150 However, a structural MRI study in adult females who experienced childhood sexual abuse revealed hippocampal shrinkage.151 According to recent studies, there is some consensus that the effects of early developmental stress on the hippocampus may be masked during childhood and may not become evident until late adolescence and adulthood.139,140,152 Thus, the effects of stress in early developmental periods on the hippocampus appear to be crucially dependent on the developmental age when the neuroimaging measures are performed.
Effects of Chronic Stress on Corticolimbic Pathways
The amygdala and hippocampus have strong bidirectional projections with the PFC.153,154 Given that stress profoundly impacts these individual brain regions, it is expected that stress would alter the crosstalk between these areas. For example, adolescents with a history of childhood maltreatment or trauma exhibited weaker resting state functional connectivity (rs-fc) between PFC regions (specifically the vmPFC and ACC) and limbic regions, including the amygdala and hippocampus.155–157 Specifically, a history of childhood maltreatment altered ACC-amygdala and ACC-hippocampal resting-state connectivity in adolescent females; however, adolescent males showed deficits only in ACC-hippocampal connectivity, which may account for increased internalizing symptoms observed in female adolescents.156 The results from a longitudinal study by Burghy et al. lend further support to the hypothesis that altered PFC-amygdala connectivity may underlie future problems with emotion regulation. Specifically, stress experienced at the age of 4 was associated with increased cortisol in childhood, which predicted lower amygdala-vmPFC functional connectivity 14 years later in females. Further, amygdala-vmPFC connectivity was associated with depressive symptoms.82 Additional validation for the role of aberrant corticolimbic connectivity comes from a recent longitudinal study by Barch et al. This study showed that poverty in early childhood is associated with abnormal hippocampal/amygdala functional connectivity with the PFC, which is further linked to negative mood symptoms.158 Overall, these studies provide evidence that stress during development may increase the likelihood of internalizing problems during adolescence, which may be mediated by abnormal corticolimbic connectivity.
Adolescents exposed to chronic stress also have demonstrated aberrant connectivity in corticolimbic networks while performing behavioral tasks. For example, while viewing negative faces, children and adolescents exposed to adverse rearing conditions during infancy displayed a mature pattern of amygdala-prefrontal connectivity (negatively coupled) as compared to controls that showed an immature pattern (positively coupled).159 In agreement, during an aversive learning paradigm, previously institutionalized children and adolescents showed adult-like patterns of amygdala-vmPFC functional connectivity, suggesting that early life stress may accelerate development of the prefrontal-amygdala pathway.160 Further, during an emotional-conflict task, adolescents with a history of childhood trauma failed to dampen dlPFC activity and engage amygdala-cingulate inhibitory circuitry, indicative of poor affect regulatory function.161 In conclusion, chronic stress during development causes morphological, structural, and functional changes in the PFC, hippocampus, and amygdala at a substrate and circuit level, which may have behavioral implications for emotion, affect, and fear regulation given the importance of corticolimbic circuits in these functions.
Effects of Chronic Stress on Neurochemical and Metabolic Profile
Chronic stress causes significant changes in neurotransmission and neural metabolites, which may provide a molecular basis for emergence of psychopathology. For example, chronic unpredictable stress in adolescence resulted in long-lasting changes in monoamine levels evident in adulthood in mice. Specifically, serotonin (5-HT) levels in the cortex, hippocampus, and hypothalamus decreased and norepinephrine (NE) levels in the hippocampus and hypothalamus decreased after chronic stress.162 A number of studies have consistently shown changes in the dopaminergic system in the PFC of adult rats, such as reduced basal dopamine (DA), decreased expression of D2 receptors, increased dopamine transporter (DAT) binding, in response to chronic stress during adolescence.163–165 Positron emission tomography (PET) is useful in probing functional neurochemistry in humans; however, PET studies in chronic stress-exposed adolescents are scarce. Adults with trauma exposure during adolescence showed reduced 5-HT1B receptor expression in the ACC and amygdala as compared to healthy controls, irrespective of PTSD diagnosis, suggesting that early chronic stress can produce long-lasting dysregulation of the 5-HT system.166 Dysregulation of the monoaminergic system in response to chronic stress experienced during adolescence has important implications for stress-induced psychopathology given the central role of monoaminergic neurotransmission in mood disorders and SUDs.12
Magnetic resonance spectroscopy (MRS) has been used increasingly in preclinical and clinical studies to investigate in vivo neurochemistry. Rats exposed to maternal stress showed reduced N-acetylaspartate (NAA) levels in the PFC during adolescence, suggesting reduced neuronal integrity and viability.167 Interestingly, a stress-induced reduction in prefrontal glutamate levels was observed in female but not male adolescent rats. This evidence has significant clinical implications for three reasons. First, reduced NAA has been observed in human adolescents with treatment-resistant depression.168 Second, dysregulated glutamatergic neurotransmission has been implicated in the pathophysiology of stress-related neuropsychiatric disorders in preclinical and clinical studies.169–171 Lastly, females have higher rates of depression,172 and the fact that they show reduced glutamate levels in response to stress while males do not highlights sex-specific neurobiological vulnerabilities for depression. Human MRS results are in agreement with those observed in rodent studies. Adolescents and children with PTSD secondary to maltreatment showed reduced NAA levels in the ACC.173Together, these studies suggest long-lasting and widespread stress-induced changes in neurotransmitter and metabolite levels, which may contribute to psychopathology in adolescence and adulthood. Thus, stress interacts with all levels of units of analysis from molecules to circuits in the RDoC matrix (Tables 1 and 2).
Impact of Chronic Stress on Behavior: Focus on Anhedonia
The neurobiological effects of stress delineated so far are bound to have behavioral ramifications given that circuits affected by stress modulate different aspects of behavior. We focus specifically on anhedonia due to its association with chronic stress and prevalence in adolescence.10,11 Moreover, anhedonia is characterized by dysregulation of circuits involving the PFC, amygdala, and hippocampus, regions that are notably affected by chronic stress (for review, see Sternat and Katzman174), and implicated in reward processing. However, a similar case can be made for other behaviors like fear extinction.
Anhedonia, defined as the state of reduced ability to experience feelings of pleasure in response to previously pleasurable or rewarding stimuli, is associated with a number of neuropsychiatric disorders including depression and SUD.174–176 Anhedonia is reflective of deficits in reward processing and has been shown to be associated with chronic stress.177 Chronic mild stress in adult animals caused reduced intake of palatable liquids (anhedonic behavior), which lasted up to one month after cessation of the stress.178,179Chronic social stress and early maternal deprivation also have been associated with anhedonia-like behaviors in adolescent and adult rodents,180–183 suggesting that chronic stress is associated with anhedonia across different stages of the lifespan, at least in rodent models.
Human studies have linked stress to anhedonia in adolescents. For example, sexual abuse between the ages of 7 and 12 was associated with behavioral and neural deficits in reward learning, one of the features of anhedonia in adulthood.184 Further, young adults with maltreatment during childhood reported elevated anhedonia and rated rewarding stimuli less positively.185 Similarly, adolescents who suffered childhood maltreatment exhibited elevated anhedonic symptoms.186,187 Another study in adolescents demonstrated that perceived stress interacts with genotype to influence reward responsiveness, a core component of anhedonia.188 Blunted responsiveness to rewarding stimuli may increase the risk for depression and compensatory reward-seeking may increase the risk for maladaptive behaviors like substance abuse.189 These studies suggest that chronic stress in adolescents causes anhedonia, which may confer vulnerability for psychopathologies.
Neurobiological Substrates of Anhedonia
Stress-induced dysregulation in corticolimbic circuits may drive stress-induced anhedonia, described in the previous section. Indeed, studies investigating the neurobiological basis of anhedonia have consistently found involvement of the vmPFC, the OFC, and the ACC, regions critical for emotion regulation.175 For instance, a fMRI study by Harvey et al.190 reported a positive correlation between anhedonia severity and vmPFC activity while processing positive stimuli in non-clinical adult subjects. Further support for involvement of the ACC in anhedonia comes from a recent study in depressed adolescents reporting that anhedonia severity is associated with alterations in intrinsic functional connectivity between the striatum and the ACC.191 In a MRS study of adolescents, anhedonia severity was negatively correlated with GABA in the ACC,192 suggesting that dysfunctional GABAergic transmission in the ACC may contribute to anhedonia severity. Reduced responsiveness of the amygdala to positive stimuli is associated with anhedonic symptoms in adult depressed patients,193 which may be related to its importance in attributing salience to environmental stimuli and production of affective states. The hippocampus also has been linked to stress-induced anhedonia. For example, anhedonia in adolescence associated with postnatal maternal separation is linked to altered hippocampal transmission.194 Overall, these studies suggest that aberrant corticolimbic crosstalk may provide a neurobiological basis for stress-induced anhedonia.
From a neurotransmitter perspective, anhedonia is associated with dysfunction in DA transmission,175 which is consistent with the central role that DA plays in the reward system.195 The mesocorticolimbic pathway drives approach behaviors and mediates positive reinforcement and learning.196 Further, glutamate is a critical metabolite in hedonic processing with activation of glutamate receptors shortening the reaction time to stimuli predictive of reward.197 5-HT modulates DA release and hence also has been shown to play a regulatory role in reward processing.198 These studies suggest that stress-induced dysfunction in DA, glutamate, and 5-HT transmission may be underlying neurochemical mechanisms (although not exclusive) in stress-induced anhedonia. Thus, the effects of stress on neural structures and neurotransmission may cause dysregulation of the reward circuit leading to anhedonia. This is in line with the fundamental tenet of RDoC that states that there exists a relationship between biology (e.g., circuits) and behaviors.
Cutting Across Diagnostic Boundaries: Depression and SUD Risk in Adolescence Share Neurobiological and Behavioral Commonalities
Adolescence is a time in development wherein a number of psychiatric illnesses emerge.24 Depression and SUD are highly prevalent in adolescence and frequently present comorbidity, with the presence of both diagnoses related to more serious consequences, such as higher risk of suicide, greater impairment, and poorer prognosis than either diagnosis alone.13,199–203 The high comorbidity of depression and SUD raises the important question of whether the two disorders are different symptomatic expressions of similar neurobiological abnormalities.4,12 An important bridging construct between depression and SUD is the preeminent role of chronic stress in the development of these disorders.4 The following sections elucidate the transdiagnostic biobehavioral processes in depression and SUD, considering chronic stress as an important environmental vulnerability factor, with an overall goal of identifying homogenous targets for treatment intervention.
Corticolimbic Circuit Dysfunction in Depression and SUD
Aberrant corticolimbic connectivity, measured by DTI and fMRI techniques, has been consistently linked to depression and SUD in adolescence. For example, a DTI study in depressed adolescents showed reduced fractional anisotropy (FA) in the uncinate fasciculus (UF), which connects the ACC and OFC to amygdala, implying reduced structural connectivity between these regions.204 Further, the rs-fc between amygdala, and vmPFC and dlPFC was reduced in adolescent depression.205 Together, these studies suggest that reduced cortical-amygdala structural and functional connectivity may be the pathophysiology underlying adolescent depression. In addition, the rs-fc between the amygdala and several other regions (e.g., hippocampus, brainstem) is reduced in adolescent depression.206 With regard to the role of hippocampus, adolescent depression was associated with lower hippocampal volumes as well as reduced connectivity between the PFC and hippocampus.207–209 For example, using a combination of DTI and rs-fMRI techniques, Geng et al.209 showed compromised structural and functional PFC-hippocampus connectivity in first-episode medication naïve adolescent depression. Further, adolescent depression is also characterized by aberrant functional crosstalk of the PFC with the striatum, a critical limbic region for reward processing. For instance, a task-based fMRI study showed altered activation of different PFC regions, including ACC and OFC and the striatum in response to reward in depressed adolescents.210 Finally, depressed adolescents exhibited altered task-based and rs-fc between different cortical regions.211–213 We note that resting state and task-based connectivity studies have reported increased as well as decreased corticolimbic connectivity in adolescent depression. It is hypothesized that decreased connectivity could reflect ineffective recruitment of the PFC to manage amygdala activity while increased connectivity may reflect prolonged and persistent experience of negative emotion.214 Together, these studies implicate altered corticolimbic crosstalk in adolescent depression.
Neuroimaging studies have demonstrated that corticolimbic dysregulation can increase risk for SUD. Studies that investigate neurobiological vulnerability factors in high-risk drug-naïve youth to eliminate the potential confounds of effects of drugs of abuse on brain function are of particular importance. Smaller OFC volumes at age 12 predicted initiation of cannabis use by age 16, suggesting structural abnormalities in the PFC, specifically the OFC, are a risk factor for initiation of cannabis use.215 Concordantly, thinner and smaller prefrontal regions, particularly the dlPFC, were associated with future binge drinking in adolescents.216 Reduced amygdala volume in adolescents was observed with a family history of alcohol dependence, implying that smaller amygdala volume could be a pre-existing risk factor for alcohol abuse.217–220 Lower hippocampal volumes were consistently observed in adolescents with alcohol use disorder;221,222 however, high-risk (HR) alcohol-naïve adolescent males demonstrated larger hippocampal volumes than controls.223 These results suggest that lower hippocampal volume may be a consequence of heavy alcohol use rather than a risk factor that predates alcohol use. In addition to these volumetric findings, DTI and rs-fMRI studies showed altered white matter integrity and rs-fc between the OFC and ventral striatum in HR substance-naïve adolescents.224–226 Task-based fMRI studies demonstrated altered patterns of activation in the corticostriatal network during risky decision-making and reward receipt in HR adolescents.227,228 Specifically, HR adolescents showed increased striatum, ACC and vmPFC activation with increasing risk of negative outcome compared to low-risk adolescents.227 Elevated activation of these regions was also observed during anticipation of reward-receipt in HR adolescents.228 In addition, abnormalities in activation of key PFC regions have been seen during facial emotion processing and response inhibition in HR adolescents.229,230 Further, adolescents at HR for SUD by virtue of having one parent diagnosed with SUD exhibited left amygdala hyperactivity in response to viewing affect-laden faces, indicative of emotion dysregulation.231 In conclusion, there is converging evidence to support a role for abnormalities in corticolimbic networks, such as the PFC-amygdala, PFC-hippocampus, PFC-striatum, as a transnosological mechanism underlying depression and risk for SUD in adolescents.
From a neurochemical perspective, there are similar alterations in several neurotransmitters that are associated with depression and substance use (for review, see Marko et al.12). For example, it has been hypothesized that reduced 5-HT levels mediate depression, which is also seen during withdrawal from several drugs of abuse. Dysfunction in DA transmission has been associated with both depression and substance use, with some arguing that low DA levels in depression drive individuals to “self-medicate” drugs of abuse. Further, it has been suggested that glial-cell-mediated glutamatergic dysfunction may contribute to the comorbidity.232
Anhedonia in Depression and Substance Use
Anhedonia is considered a core symptom, and a major risk factor and potential trait marker, for depression.233 In adolescents, anhedonia is considered to be an indicator of poor prognosis because it is associated with longer time to remission and fewer depression-free days.234 Anhedonia also has been associated with initiation and escalation of substance use in adolescents. Adolescents low in hedonic capacity were over two-and-a-half times more likely to have smoked a cigarette in the past month at age 15 and showed a 90% increase in smoking escalation over the next 18 months compared with adolescents with high hedonic capacity.235,236 Interestingly, in adolescents who had never smoked, those with higher anhedonia reported greater expectancies that smoking caused pleasure, suggesting that anticipated pleasure from smoking in anhedonic non-smoker adolescents may confer an initiation risk.237 Although there is evidence that anhedonia was positively associated with lifetime psychostimulant use and transition to dependence in adults,238 whether the association between anhedonia and psychostimulant use holds true in adolescents needs to be investigated. Together, these studies provide evidence that anhedonia is a common behavior observed in depression and initiation and escalation of substance use in adolescents.
Discussion
Future Directions
Despite substantial extant literature on the effects of chronic stress, additional investigations are needed to characterize the impact of stress during adolescence. First, the sex difference in stress-induced vulnerability to psychopathology during this developmental period requires further study. Interestingly, females who have been exposed to developmental stress are more likely to develop depression than males.172 Further, females are twice as likely as males to develop depression and also have higher levels of anxiety; this difference does not emerge until puberty, suggesting that sex differences in psychopathology may play an important role in adolescence.239 There also are sex differences with regard to the timing and trajectory of development of corticolimbic circuits during adolescence (for review, see Hammerslag and Gulley240). The complex interactions between sex and development may be an important factor contributing to the sex differences in stress-induced psychopathologies during adolescence. Second, further research into the stress-generation and diathesis-stress theories, which suggest that pre-existing individual vulnerabilities may predispose a person to a stress-induced psychopathology,241 is vital. Third, the contribution of gene-environment interactions to stress-induced psychopathology needs to be investigated. For example, a recent study in adults showed that perceived chronic stress interacts with genotype to increase susceptibility of stress-induced psychopathology.242 Future studies investigating factors influencing adolescent vulnerability to stress should include sex and genetic predispositions, which will help clarify differences in disease vulnerability. Further, most of the studies investigating effects of chronic stress are cross-sectional in design. Alterations in neurobiology reported due to chronic stress may have existed prior to the onset of stress, reflecting a risk/vulnerability factor rather than a consequence of stress. A longitudinal study design may reveal interactions with temporal changes in brain connectivity, metabolite concentration, and other brain-based measures, which have been shown to change with developmental stage.
An important consideration while interpreting the effects of stress on neurobiology is the heterogeneity of stress, especially in human studies. Stress effects may be different depending on the nature and timing of the stressor. Some of the divergent findings in human studies can be attributed to the uncontrollability of the temporal parameters of the stressor. For example, human studies include temporally diverse types of stress: chronic stress throughout development, stress limited to infancy or childhood, or chronic stress during adolescence, each of which may have differential effects on brain development (Figure 1).189,243,244 Further, difference in the nature of the stressor (e.g., abuse vs. poverty) and whether the stressor is present during the window of assessment are also important considerations. One study investigating whether brain regions showed differential vulnerability depending on the age of onset for chronic stress revealed that sexual abuse between the ages of 3–5 was associated with reductions in hippocampal volume, whereas sexual abuse between the ages of 14–16 was associated with reductions in PFC volume,245 suggesting that stress-induced changes in different neural substrates may be aggravated or buffered depending on the timing of stress. Future human studies should investigate whether brain structures have different developmental windows (infancy vs. childhood vs. adolescence) during which they are most susceptible to influences of chronic stress (Tables 1 and 2).
Heuristic Model
Based on the findings described above, a heuristic model is suggested that appears to underlie a number of stress-induced changes (Figure 3). The model posits that dysregulation of corticolimbic circuits along with deficits in DA, 5-HT, and glutamate transmission may underlie independent diagnostic entities in adolescence. Alterations of the same biological circuitry contribute to transnosological behavioral domains, such as anhedonia that comprise distinct DSM diagnoses, depression and SUD. The model is complicated by inclusion of environmental and developmental influences, each of which may have bidirectional complex interactions with neural circuits, behavior, and diagnosis. Overall, this model proposes that chronic stress during development has profound effects on the ontogeny of neural circuits, both at an anatomical and molecular level. Stress-induced modifications in key brain networks may then cause dimensional changes in behavioral domains ultimately increasing the risk of psychopathologies. Using an example of depression and SUD, we hypothesize that seemingly distinct disorders may have similar neurobiological and behavioral signatures. Hence, treating the disorder only on the basis of the presenting signs and symptoms may not be adequate. Instead new approaches will need to target the underlying neurobiological pathophysiology. Based on the model, we propose that interventions that amend dysregulated corticolimbic circuits and rescue anhedonic behaviors may provide better prognostic outcomes in adolescents suffering from depression and SUD. Finally, chronic stress and development interact with all units of analysis of the RDoC matrix affecting molecules, circuits and behaviors. In light of this interaction, integration of environment and development in the RDoC matrix will strengthen the framework and enable a better understanding of their influence on the emergence of psychopathology.
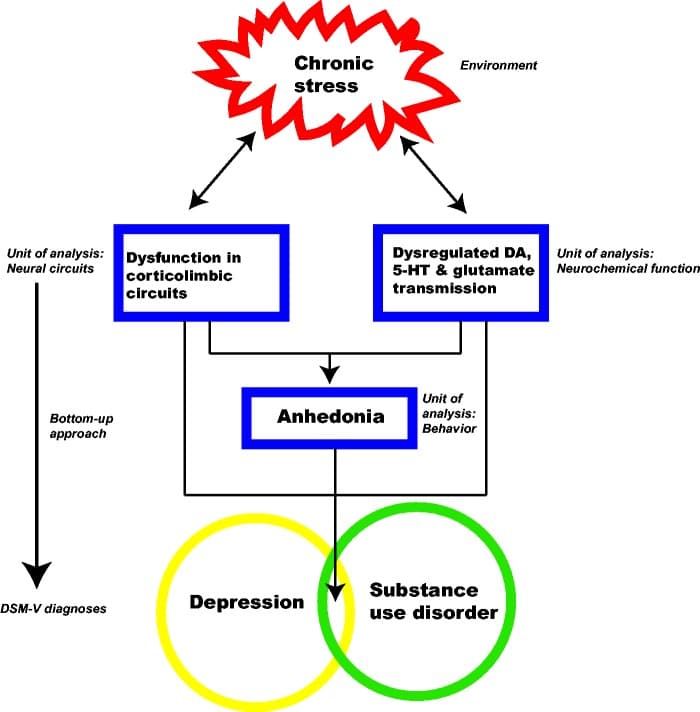
Figure 3. A model showing interactions of chronic stress (environment) with neurobiology and behavior in adolescence, which may increase risk for comorbid disorders such as depression and SUD.
Declaration of Conflicting Interests
The author(s) declared no potential conflicts of interest with respect to the research, authorship and/or publication of this article.
Funding
The author(s) disclosed receipt of the following financial support for the research, authorship, and/or publication of this article: This research is supported by the Utah Science Technology and Research Initiative (USTAR); the Adolescent Brain and Cognitive Development Consortium U01DA041134; and the Department of Veterans Affairs Rocky Mountain Network Mental Illness Research, Education, and Clinical Center (MIRECC). The views in this paper are those of the authors and do not necessarily represent the official policy or position of the Department of Veterans Affairs or the United States Government.