Abstract
An extensive literature on childhood adversity and neurodevelopment has emerged over the past decade. We evaluate two conceptual models of adversity and neurodevelopment—the dimensional model of adversity and stress acceleration model—in a systematic review of 109 studies using MRI-based measures of neural structure and function in children and adolescents. Consistent with the dimensional model, children exposed to threat had reduced amygdala, medial prefrontal cortex (mPFC), and hippocampal volume and heightened amygdala activation to threat in a majority of studies; these patterns were not observed consistently in children exposed to deprivation. In contrast, reduced volume and altered function in frontoparietal regions were observed consistently in children exposed to deprivation but not children exposed to threat. Evidence for accelerated development in amygdala-mPFC circuits was limited but emerged in other metrics of neurodevelopment. Progress in charting neurodevelopmental consequences of adversity requires larger samples, longitudinal designs, and more precise assessments of adversity.
Introduction
Adverse environmental experiences in childhood—such as exposure to violence, abuse, neglect, separation from caregivers, and chronic poverty—have powerful and lasting influences on children’s development. Altered patterns of emotional, cognitive, and social development have been observed consistently among children who have experienced adversity. Substantial evidence also indicates that adverse childhood experiences influence neural development, leading to changes in the pace and progression of neural development across metrics of brain structure, function, and connectivity. Identifying the neurodevelopmental mechanisms that link experiences of adversity with physical and mental health, school achievement, and social and economic functioning is critical for developing more effective early interventions. Here, we focus on neurodevelopmental pathways that might contribute to these long-term disparities. Specifically, we provide the first systematic review of the existing literature on childhood adversity and neural development.
Defining Childhood Adversity
Despite the substantial body of research examining the developmental consequences of childhood adversity, there has been a surprising lack of consistency with regard to the definition and measurement of the construct. We use the term childhood adversity to refer to negative environmental experiences that are likely to require significant adaptation by an average child and that represent a deviation from the expectable environment (McLaughlin 2016). This definition builds upon modern definitions of life stress (Monroe 2008) as well as models of experience-expectant brain development (Fox et al. 2010). In these models, the expectable environment refers to a wide variety of experiences that the human brain expects to encounter to develop normally. These include social experiences such as the presence of a sensitive and responsive caregiver as well as sensory and perceptual experiences; for example, light input to the eye is required for the visual system to develop (Fox et al. 2010). Adverse childhood experiences reflect deviations from this expectable environment and can involve the presence of an unexpected experience that represents a significant threat of harm to the child, such as exposure to violence or abuse, or the absence of some type of experience or environmental input the brain expects to develop normally, such as the lack of a primary caregiver or limited exposure to language (Humphreys & Zeanah 2015, McLaughlin & Sheridan 2016, McLaughlin et al. 2014a, Sheridan & McLaughlin 2014). To qualify as adversity, experiences of threat or deprivation must either be chronic (e.g., prolonged separation from a caregiver) or involve single events that are severe enough to require significant emotional, cognitive, or neurobiological adaptation by an average child (e.g., sexual abuse).
Scope of the Problem
Exposure to childhood adversity is common. Population-based studies indicate that approximately half of all children will experience at least one form of adversity by the time they reach adulthood in the United States and cross-nationally (Green et al. 2010, Kessler et al. 2010, McLaughlin et al. 2012). Children who experience adversity are about twice as likely to develop a mental disorder as those who have not, and the odds of developing psychopathology increase dramatically as exposure to adversity increases (Lewis et al. 2019, McLaughlin et al. 2012). Increased risk for psychopathology among those who have experienced childhood adversity persists throughout adolescence and adulthood, and approximately one-third of all mental disorders worldwide are attributable to exposure to childhood adversity (Green et al. 2010, Kessler et al. 2010, McLaughlin et al. 2012). These types of adverse experiences are also associated with poor academic achievement (Lansford et al. 2002), reduced social and economic functioning (Zielinski 2009), numerous chronic diseases and leading causes of death (Felitti et al. 1998), and even early mortality (Chen et al. 2016).
Conceptual Models of Adversity and Neural Development
The prevailing approach to conceptualizing adversity over the past decade has been the cumulative risk approach. This approach tallies the number of distinct forms of adversity a child has experienced to create a risk score without regard to the type, chronicity, or severity of the experience and uses this risk score as a predictor of outcomes (Evans et al. 2013). For example, a child who experienced physical abuse, sexual abuse, and domestic violence would have a risk score of three; a child who experienced poverty, neglect, and maternal depression would also have a risk score of three. Cumulative risk thus focuses on the number of distinct types of adversity a child has experienced rather than the severity of those experiences or type of adversity. It is assumed that the effects of these different environmental experiences are not only qualitatively similar but also quantitatively similar, such that each distinct type of adversity will have an effect of equal magnitude on any developmental process in question (Evans et al. 2013). Disruptions in stress response systems and other regulatory systems associated with allostatic load represent the common mechanism through which various forms of seemingly disparate adverse experiences are thought to influence the wide variety of developmental outcomes associated with adversity in cumulative risk models (Evans & Kim 2007, Evans et al. 2013). Neurodevelopmental pathways through which adversity might influence health and other outcomes have rarely been articulated in cumulative risk models. As such, we focus our review on more recent conceptual models of adversity that focus specifically on these neurodevelopmental mechanisms.
Dimensional Model of Adversity
In contrast to the cumulative risk approach, the dimensional model of adversity posits that adversity exposures reflect multiple underlying dimensions of environmental experience that may have distinct associations with neurodevelopmental processes (McLaughlin & Sheridan 2016, McLaughlin et al. 2014a, Sheridan & McLaughlin 2014). This model is based on two principles. First, it is possible to identify core underlying dimensions of environmental experience that occur in numerous types of adversity that share common features. Two initial dimensions proposed in this model are threat—encompassing experiences involving harm or threat of harm to the child—and deprivation, which involves an absence of expected inputs from the environment during development, such as support, nurturance, and cognitive and social stimulation. These dimensions underlie numerous experiences that reflect the core features of threat and deprivation to varying degrees. For example, physical and sexual abuse, witnessing domestic violence, and exposure to violence in the community all involve threat of harm to the child to varying degrees. In contrast, deprivation involving low levels of social and cognitive stimulation is a core feature of neglect, institutional rearing, and other forms of parental absence and occurs more often in families with low socioeconomic status (SES), although not universally (Bradley et al. 2001).
The second core principle of the model is that different dimensions of adversity have distinct influences on cognitive, emotional, and neural development. Although some neurodevelopmental processes may be influenced broadly by multiple forms of adversity, others may be uniquely influenced by particular types of early experiences (McLaughlin & Sheridan 2016). As such, it is expected that the neurodevelopmental processes influenced by experiences of threat are at least partially distinct from those influenced by deprivation. Specifically, this model argues that exposure to violence during periods when neural systems that support learning about threat and safety are highly plastic will alter these circuits in ways that facilitate the rapid identification of threats in the environment—an adaptive response to being raised in an environment characterized by danger. Experiences of threat are expected to have strong influences on neural systems that are involved in threat detection and learning, salience processing, and emotion regulation (McLaughlin et al. 2014a, Sheridan & McLaughlin 2014). These include what is often referred to as the limbic (Yeo et al. 2011) or fronto-amygdala system (Tottenham 2015), comprising the amygdala and medial prefrontal cortex (mPFC), as well as the hippocampus. Behavioral studies are consistent with these predictions, demonstrating changes in emotion perception, attention to emotional stimuli, aversive learning, emotional reactivity, and emotion regulation in children exposed to violence (Heleniak et al. 2016, McLaughlin et al. 2016, Pollak & Sinha 2002, Pollak & Tolley-Schell 2003), particularly for negative stimuli that signal the presence of threat (McLaughlin & Lambert 2017).
In contrast, experiences of deprivation that involve reductions in cognitive and social stimulation—such as neglect and caregiver deprivation—are posited to have particularly pronounced influences on cognitive development, including language, executive functioning, and other aspects of higher-order cognition. Children who experience less frequent and stable caregiving are deprived not only of support and nurturance but also of a diversity of sensory, motoric, linguistic, and social experiences that are critical for early learning. This type of deprivation may alter neural development through multiple mechanisms, including synaptic pruning. Pruning is a primary driver of experience-dependent plasticity that produces a neural system that is maximally efficient for the environment in which it developed (Holtmaat & Svoboda 2009). Reduced exposure to inputs that the brain expects to experience, particularly during sensitive periods, can lead to accelerated and exaggerated pruning in the neural circuits that process these types of inputs (Hensch 2005), producing reductions in cortical thickness and volume and changes in neural function within these circuits that may ultimately contribute to differences in a variety of complex social and cognitive abilities (McLaughlin et al. 2017). Indeed, difficulties with language and executive functions among children exposed to early deprivation are well established (Pollak et al. 2010, Spratt et al. 2012); these differences are mediated by the degree of cognitive and social stimulation in the early environment (Rosen et al. 2019, Sarsour et al. 2011). In addition to these behavioral differences, the dimensional model argues that altered structure and function in the neural networks that support these complex cognitive abilities will also be observed, particularly in the frontoparietal network that supports executive functions (McLaughlin et al. 2014a, 2017; Sheridan & McLaughlin 2014). These differences in frontoparietal structure and function are not expected to emerge following threat exposure, although alterations in emotional processing associated with threat may contribute to difficulties with cognitive control in emotionally salient contexts. Existing data are consistent with this idea (Lambert et al. 2017a).
The primary predictions of the dimensional model involve circuits contributing to salience processing, aversive learning, and emotion regulation—which are argued to be primarily influenced by threatening early environments—and frontoparietal circuits that underlie cognitive control, which are thought to be primarily influenced by deprivation. Multiple models of adversity also argue that development of the frontostriatal network involved in reward-related processing will be influenced by experiences of adversity (McCrory et al. 2017a, McLaughlin & Sheridan 2016), with experiences of deprivation and those involving a lack of contingent responding to the child particularly likely to produce blunted responses in this network during the anticipation and receipt of rewards (Sheridan 2017).
Importantly, the dimensions of threat and deprivation encompass many types of adverse experiences but certainly not all, and other dimensions of experience (e.g., unpredictability) are clearly important in shaping neurodevelopment. Childhood poverty or low SES is one example of a potential form of adversity that does not map clearly onto either of these dimensions. Within the dimensional model, poverty is conceptualized as a context that increases risk for exposure to threat (e.g., neighborhood violence), cognitive and material forms of deprivation (e.g., reduced exposure to language and cognitive stimulation, food insecurity), and other adverse experiences (e.g., crowding, exposure to toxins). Given the multifaceted nature of adversity experiences among children raised in poverty, we do not include studies focused explicitly on SES as indicators of either threat or deprivation in this review.
Stress Acceleration
A second conceptual model argues that adversity might accelerate the pace of neural development, leading to faster maturation in neural circuits underlying emotional processing (Callaghan & Tottenham 2016a,b). This model is based on the notion that the presence of a supportive caregiver during a sensitive period in early development buffers children’s emotional and neurobiological responses to stress, thereby scaffolding emotional learning while the fronto-amygdala circuits that underlie emotional processing develop. When children are raised in the absence of a sensitive and responsive caregiver—the cardinal feature of institutional rearing and neglect but also common in children exposed to abuse and other forms of maltreatment—the development of fronto-amygdala circuits accelerates, potentially to compensate for the absence of species-expectant maternal buffering of emotional reactivity (Callaghan & Tottenham 2016b). While it is potentially adaptive in the short term for this circuit to develop more quickly in the absence of a caregiver to promote emotion regulation skills, the premature closure of the sensitive period for caregiver influences on emotional learning may lead to suboptimal emotion functioning in the long term.
A specific hypothesis of the stress acceleration theory is that connectivity between the amygdala and mPFC matures more quickly in children raised in the absence of supportive or consistent caregiving (Callaghan & Tottenham 2016b). In other words, children exposed to caregiver deprivation should exhibit patterns of amygdala-mPFC connectivity that resemble those of adolescents or young adults who have not experienced this form of adversity. If this model is correct, this should be true for both structural and functional amygdala-mPFC connectivity.
Systematic Review of Childhood Adversity and Neural Development
Here, we perform a systematic review of studies examining associations of childhood adversity with brain structure, function, and connectivity in children and adolescents. Multiple reviews have been conducted on this topic, although we are unaware of any systematic reviews. Many reviews focused on a limited number of adverse experiences, such as child maltreatment (McCrory et al. 2010,2017a; Teicher et al. 2016) and institutional rearing (Bick & Nelson 2016), or a limited set of brain areas (Tottenham & Sheridan 2010). Others have included neuroimaging studies conducted in adult participants that rely on retrospective reporting of adversity that often occurred decades earlier (Hein & Monk 2016, Lim et al. 2014, Teicher et al. 2016).
We structure our review to evaluate the hypotheses of two leading conceptual models of adversity and neural development. We take a broader view on adversity than prior reviews, including studies examining multiple forms of threat—including physical, sexual, and emotional abuse, exposure to domestic violence, and exposure to other forms of violence, as well as multiple forms of deprivation—including institutional rearing, physical and emotional neglect, and extreme material deprivation involving lack of access to basic necessities such as food or shelter. Given a recent comprehensive review of SES and neural outcomes (Farah 2017) and the fact that SES does not clearly reflect either threat or deprivation, we do not include studies focused exclusively on SES.
An explicit goal of this review is to systematically evaluate how experiences of threat and deprivation are associated with neural development (McLaughlin et al. 2014a); as such, we review findings separately for these dimensions of adversity. However, because many prior studies have not conceptualized adversity in this way—often combining multiple distinct forms of adversity together—we also review findings from samples with mixed adversity exposures. We additionally evaluate the predictions of the stress acceleration model, arguing that childhood adversity accelerates neurodevelopment in circuits underlying emotional processing (Callaghan & Tottenham 2016a,b). We focus on studies in which neuroimaging measures were collected during childhood and adolescence, given a recent meta-analysis demonstrating that retrospective measures of adversity collected in adulthood identify different individuals than prospective measures collected during childhood (Baldwin et al. 2019), highlighting difficulties in comparing studies of children and adults.
We organize our review around the following brain networks: fronto-amygdala (often referred to as the limbic network in cortical network discovery studies) (Power et al. 2011, Yeo et al. 2011), salience, hippocampal-prefrontal, frontoparietal, and frontostriatal circuits. We focus specifically on MRI studies, which comprise the majority of neuroimaging studies of childhood adversity. We include MRI studies examining neural structure, neural function in response to specific cognitive and emotional tasks, and neural connectivity, including resting-state functional connectivity (rs-fc), task-based functional connectivity, and diffusion tensor imaging measures of white matter microstructure. To accomplish these goals, we searched for all papers examining differences in neural structure, function, or connectivity among children, adolescents, or both as a function of adversity exposure. Details on our search terms, search strategy, and inclusion and exclusion criteria are provided in Supplemental Text and Supplemental Table 1. Supplemental Figure 1 summarizes the number of papers screened, excluded, and included in the final review. A total of 109 empirical papers were included. Of those, nine used methods for analyzing structural or functional connectivity—typically graph theory methods—that were difficult to integrate with other papers and were not quantitatively reviewed. A list of those studies is provided in the Supplemental References.
Fronto-Amygdala Circuits and Threat Processing
A circuit comprising the amygdala and mPFC has been the most frequent target in neuroimaging studies of childhood adversity. This is unsurprising, given the central role of the amygdala in aversive learning, threat detection, and the mobilization of defensive responses to external stimuli (LeDoux 2003, 2012), although it is well established that the amygdala responds not only to threatening cues but also to a wide variety of stimuli that have motivational salience (Costafreda et al. 2008, Fusar-Poli et al. 2009). The amygdala has bidirectional structural connections with multiple areas of the mPFC, including the ventromedial PFC (vmPFC) and subgenual (sgACC), pregenual (pgACC) (sometimes referred to as rostral ACC), and dorsal (dACC) regions of anterior cingulate cortex (Dixon et al. 2017, Ghashghaei et al. 2007, Kim et al. 2011); we review the dACC separately in the section below titled Salience Network. The amygdala and mPFC exhibit positive connectivity at rest in adults (Roy et al. 2009), and this positive coupling increases from childhood to adulthood (Gabard-Durnam et al. 2014). Task-specific changes in the strength of amygdala-mPFC connectivity are also consistently observed during emotion regulation and threat processing tasks, particularly with the pgACC and vmPFC (Di et al. 2017). As we review below, alterations in amygdala structure, function, and connectivity with the mPFC have been reported frequently among children exposed to adversity; see Figure 1 and Supplemental Table2 for details on all included studies.
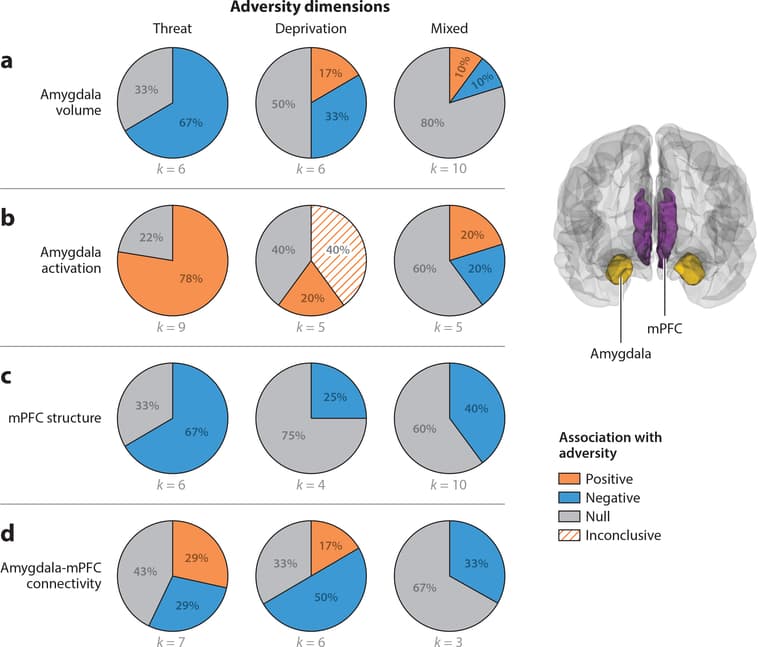
Figure 1: Pie charts represent the proportion of studies that find positive (orange), negative (blue), or null (gray) associations between each adversity type and (a) amygdala volume, (b) amygdala activation, (c) medial prefrontal cortex (mPFC) structure, and (d) amygdala-mPFC connectivity. Studies of mPFC structure include those that used measures of cortical thickness, volume, or surface area. Amygdala activation refers to studies that contrasted negative emotional stimuli with neutral emotional stimuli. For two of the five studies of the relation between deprivation and amygdala activation, fearful faces were contrasted against the implicit baseline of the intertrial interval instead of a neutral stimulus. Studies of amygdala-mPFC connectivity summarized in panel d include studies measuring either fractional anisotropy of the uncinate fasciculus or seed-based resting-state functional connectivity of the amygdala.
Amygdala Volume
Figure 1a summarizes associations of threat, deprivation, and mixed exposures with amygdala volume.
Threat Of six studies reporting associations between childhood violence exposure and amygdala volume, four (67%) observed smaller amygdala volume among children and adolescents exposed to violence than among youths never exposed to violence (Hanson et al. 2015b, McLaughlin et al. 2016, Saxbe et al. 2018; D.G. Weissman, H.K. Lambert, AM. Rodman, M. Peverill, M.A. Sheridan & K.A. McLaughlin, manuscript under review), and two reported no association (Gold et al. 2016, Marusak et al. 2015b).
Deprivation Associations of deprivation with amygdala volume are mixed, with no clear pattern across studies. Early studies reported larger amygdala volume in a small sample of previously institutionalized adolescents relative to controls (Mehta et al. 2009) and a positive association between duration of institutional care and left amygdala volume but no overall effect of institutionalization (Tottenham et al. 2010). Two later studies in relatively large samples found the opposite pattern—previously institutionalized children had smaller left amygdala volume than never-institutionalized children (Hanson et al. 2015b, Hodel et al. 2015). A final study found no association between institutional rearing and amygdala volume (Sheridan et al. 2012). No differences in amygdala volume have been observed in samples of children exposed to emotional and physical neglect (Edmiston et al. 2010).
Mixed exposures Ten studies have examined amygdala volume in samples with mixed exposures, and findings are inconsistent. In the nine samples of maltreated children (combining abuse, neglect, and family violence), one reported larger left amygdala volume in maltreated children (Morey et al. 2016), seven found no maltreatment-related differences (Brooks et al. 2014, Dalvie et al. 2014, De Brito et al. 2013, Kelly et al. 2015, Liao et al. 2013, Redlich et al. 2018), and a longitudinal study observed slower growth in left amygdala during adolescence in maltreated youth (Whittle et al. 2013). A final study examining a wide variety of adversities observed reduced amygdala volume in adolescents with three or more exposures but no association in children (Korgaonkar et al. 2013).
Summary Reductions in amygdala volume have been observed most consistently among children exposed to threat but not among those exposed to deprivation or in samples with mixed exposures.
Amygdala Activation Most studies examining adversity-related differences in amygdala function have utilized functional MRI (fMRI) tasks that involve threat-related stimuli—passive viewing of negative relative to neutral stimuli (i.e., faces or social scenes depicting fear, anger, or sadness), attention orienting to threat, or aversive learning. We quantitatively summarize studies examining amygdala responses during threat processing and highlight several findings from tasks not involving threat processing. Figure 1b summarizes adversity associations of threat, deprivation, and mixed exposures with amygdala activation to negative relative to neutral stimuli.
Threat Heightened amygdala reactivity to negative emotional cues has been observed consistently among children exposed to violence. Seven of nine (78%) fMRI studies reported higher amygdala activation to negative relative to neutral cues among youths exposed to violence than those without violence exposure. This elevated amygdala reactivity has been observed in response to negative social images (McLaughlin et al. 2015; J.L. Jenness, M. Peverill, A.B. Miller, C. Heleniak, M.M. Robertson et al., manuscript under review); to angry (McCrory et al. 2011, 2013; White et al. 2019), fearful (Ganzel et al. 2013, Suzuki et al. 2014), and both sad and happy (Suzuki et al. 2014) faces relative to neutral faces; and to fearful and happy faces labeled with conflicting emotional words relative to faces with congruent labels (Marusak et al. 2015c). The two studies of threat-related stimuli that did not observe differences in amygdala reactivity in children and adolescents exposed to violence examined responses to fearful faces relative to scrambled (Weissman et al. 2019) and happy faces (Hart et al. 2018).
A final study not focused on threat processing found no violence-related differences in amygdala reactivity to neutral faces relative to objects (Marusak et al. 2015b).
Deprivation Differences in amygdala reactivity as a function of deprivation have been observed in response to fearful relative to neutral faces but not in other types of tasks. Three studies observed greater amygdala reactivity to fearful faces among children exposed to deprivation than controls. One study contrasted fearful and neutral faces (Maheu et al. 2010), but two contrasted fearful faces to implicit baseline instead of a neutral or scrambled face or other perceptual control (Gee et al. 2013a, Tottenham et al. 2011), making it difficult to conclude that differences are specific to threat-related processing. In contrast, no differences in amygdala reactivity have been found during fear conditioning (Silvers et al. 2016b) or a visual search task with fearful faces (Silvers et al. 2016a) as a function of institutional rearing.
In tasks not focused on threat processing, lower amygdala discrimination has been reported in previously institutionalized children in response to trustworthy versus untrustworthy faces (Green et al. 2016) and to pictures of one’s mother versus a stranger (Callaghan et al. 2019, Olsavsky et al. 2013).
Mixed exposures Amygdala reactivity differences have not been consistently observed in samples with mixed adversity exposures. One study found heightened amygdala reactivity to fearful relative to neutral faces in children exposed to family-related adversity (Herringa et al. 2016). In contrast, no adversity-related associations with amygdala reactivity were observed in two studies examining neural response to fearful or angry faces in maltreated children (Crozier et al. 2014, Redlich et al. 2018). A final study observed reduced amygdala activation to rejection-related relative to neutral words in maltreated children (Puetz et al. 2016).
Summary Heightened amygdala reactivity to threat cues has been observed most consistently in children exposed to threat and inconsistently in children exposed to deprivation and in samples with mixed exposures.
Medial Prefrontal Cortex Structure and Function
We focus here on the structure and function of several mPFC regions, encompassing vmPFC, sgACC, pgACC, and dorsomedial PFC (dmPFC). Figure 1c summarizes associations of threat, deprivation, and mixed exposures with mPFC structure.
Threat Reductions in the volume or thickness of vmPFC have been observed in four of six studies (67%) in children exposed to violence, including reduced vmPFC thickness (Gold et al. 2016) and volume ofvmPFC and sgACC (Butler et al. 2018, Edmiston et al. 2010, Hanson et al. 2010), with null effects in two samples (Lim et al. 2018, McLaughlin et al. 2016).
Increased mPFC responses to threat cues in children exposed to trauma were reported in three of eight studies (37.5%), including greater dmPFC activation to fearful versus neutral faces (Cisler et al. 2013) and to fearful versus happy faces (Hart et al. 2018). A final study reported increased sgACC and pgACC response to sad relative to neutral faces but no differences for anger and fear (Suzuki et al. 2014). Five studies reported no differences in mPFC recruitment to threat relative to neutral cues (Ganzel et al. 2013, McCrory et al. 2013, McLaughlin et al. 2015, Weissman et al. 2019; J.L. Jenness, M. Peverill, A.B. Miller, C. Heleniak, M.M. Robertson et al., manuscript under review).
Deprivation Only one of four studies (25%) of children exposed to deprivation reported differences in mPFC structure. Reduced volume and surface area of the pgACC were observed in previously institutionalized children (Hodel et al. 2015), with no differences in other studies of institutionally reared (Herzberg et al. 2018, Hodel et al. 2015, McLaughlin et al. 2014b) or neglected (Edmiston et al. 2010) children.None of the four studies (0%) examining neural response to threat cues observed deprivation- related differences in mPFC function, although one reported marginally increased vmPFC response to fearful faces (relative to baseline) in previously institutionalized children (Tottenham et al. 2011).
Mixed exposures In samples with mixed adversity exposures, adversity-related reductions in the volume or thickness of vmPFC were reported in four of ten samples (40%). Decreased vmPFC volume among maltreated youth was reported in two studies (De Brito et al. 2013, Kelly et al. 2015) and reduced thickness of the pgACC in three studies (Kelly et al. 2013, 2016), one of which is an overlapping sample reporting volume differences and one that observed this pattern in adolescents but not children (Korgaonkar et al. 2013). No adversity-related differences in mPFC structure were observed in six studies (Brooks et al. 2014, Dalvie et al. 2014, Liao et al. 2013, Luby et al. 2017, Morey et al. 2016, Redlich et al. 2018).One of two studies (50%) reported reduced dmPFC activation to fearful versus scrambled faces in children with mixed adversity experiences (Crozier et al. 2014), with no mPFC differences in another (Herringa et al. 2016).
Summary Reductions in the volume and thickness of the mPFC have been found consistently in children exposed to threat but not deprivation or in mixed adversity samples. Altered mPFC function during threat processing has been reported infrequently across all types of adversity experiences.
Amygdala-Medial Prefrontal Cortex Connectivity
Connectivity between the amygdala and mPFC has been examined using a variety of methods. We review papers examining the structural integrity of the uncinate fasciculus, a white matter tract that connects the temporal lobe and PFC (Von Der Heide et al. 2013), and rs-fc with an amygdala seed or using multiple nodes within the fronto-amygdala network. Finally, we describe studies of task-based functional connectivity during threat processing. Figure 1d summarizes associations of adversity exposures with amygdala-mPFC connectivity, and Figure 2 provides a summary of evidence for accelerated development in this circuit, separately for structural, resting-state, and task-based connectivity measures.
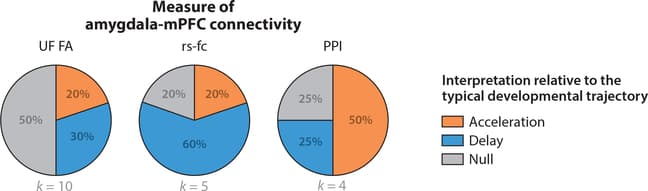
Figure 2: Pie charts represent the proportion of studies that support the stress acceleration hypothesis (orange), compared to patterns more consistent with developmental delay (blue) and null findings (gray) with regards to three measures of amygdala-medial prefrontal cortex (mPFC) connectivity across adversity types. Uncinate fasciculus (UF) fractional anisotropy (FA) typically increases across development, so higher FA would be consistent with accelerated development, while lower FA would be consistent with developmental delay. Amygdala-mPFC resting-state functional connectivity (rs-fc) typically increases across development, so greater rs-fc would be consistent with accelerated development, while lower rs-fc would be consistent with developmental delay. Task-based functional connectivity to negative versus neutral emotional cues, as measured through psychophysiological interaction (PPI) analyses, typically decreases across development, so lower task-based functional connectivity would be consistent with accelerated development, while higher connectivity would be consistent with developmental delay.
Threat One of three studies (33.3%) reported differences in uncinate fasciculus structure in children exposed to threat-related adversity. Higher fractional anisotropy (FA) in the uncinate fasciculus in children exposed to trauma was observed in a sample of approximately 1,400 participants (Gur et al. 2019), with no trauma-related differences in this tract reported in two smaller studies (Dennison et al. 2019, Park et al. 2016).
Resting-state studies of amygdala-mPFC functional connectivity in children exposed to violence have produced mixed results. One found greater rs-fc of the amygdala with sgACC and vmPFC in children exposed to violence (Thomason et al. 2015); another reported no trauma-related differences in amygdala rs-fc in a small sample of adolescents (Saxbe et al. 2018); and two papers from overlapping samples reported lower rs-fc of the right amygdala with the pgACC among adolescents exposed to violence (Cisler et al. 2013) and lower rs-fc of the left amygdala with the mPFC in adolescents who experienced emotional abuse (Cisler 2017).
Task-based functional connectivity findings are also mixed. In one study, children exposed to violence had greater negative functional coupling of the amygdala with sgACC and vmPFC to negative relative to neutral social images (Peverill et al. 2019). No trauma-related differences in amygdala-mPFC connectivity to fearful relative to scrambled faces were found in another (Weissman et al. 2019).
Deprivation Three of five studies (60%) observed reduced FA in the uncinate fasciculus in previously institutionalized children (Eluvathingal et al. 2006, Govindan et al. 2010, Kumar et al. 2013); the other two found no deprivation-related differences in this tract (Bick et al. 2015, Hanson et al. 2013). In contrast, both neglect and food insecurity were associated with higher FA in the uncinate fasciculus relative to children never exposed to adversity (Dennison et al. 2019).
An influential study found that children exhibit positive functional task-based amygdala-mPFC functional coupling when viewing fearful faces, whereas adolescents and adults exhibit negative functional coupling (Gee et al. 2013b). Previously institutionalized children exhibited negative functional connectivity of these regions when viewing fearful faces, a pattern similar to that in adolescents never exposed to adversity (Gee et al. 2013a). A final study reported positive functional connectivity between amygdala and vmPFC to a threat cue during fear conditioning in previously institutionalized children and a negative pattern of connectivity between these regions in controls (Silvers et al. 2016b). This pattern was interpreted as reflecting accelerated maturation in this circuit among youth exposed to deprivation, although no interaction of deprivation with age was found.
Mixed exposures Adversity-related differences in the uncinate fasciculus have not been observed in mixed exposure samples (Huang et al. 2012, Korgaonkar et al. 2013). Studies of amygdala-mPFC functional connectivity generally find less mature development in adversity-exposed children. One study found lower rs-fc of the amygdala and sgACC in maltreated children (Herringa et al. 2013), whereas higher task-based amygdala-mPFC functional connectivity was observed when children with mixed adversity experiences viewed fearful relative to neutral faces (Herringa et al. 2016).
Summary Although differences in amygdala-mPFC connectivity have been observed frequently in children exposed to adversity, the specific patterns vary widely across studies, with few consistent findings. Evidence for accelerated development of this circuit has generally not been observed in resting-state studies (in which higher rs-fc reflects more mature development) (Gabard-Durnam et al. 2014) or studies examining the structure of uncinate fasciculus (in which higher FA reflects greater maturity) (Lebel et al. 2012). Across adversity types, these studies largely report lower amygdala-mPFC rs-fc and FA in the uncinate fasciculus among adversity-exposed children. Evidence for accelerated development is more consistent in patterns of task-based functional connectivity during emotional processing in children exposed to deprivation but not other adversity types, although differences in the tasks and contrasts used across studies make it challenging to draw clear conclusions about these patterns.
Salience Network
Although most studies of emotional processing following adversity have focused on amygdala function, a network of regions extending beyond the amygdala responds to stimuli that have motivational salience; this network includes the anterior insula, dACC, and supplementary motor area (Seeley et al. 2007, Uddin 2015). The salience network plays a key role in coordinating activity across the default mode network—which is active during internally directed thought, including autobiographical memory and social cognition—and the frontoparietal network involved in cognitive control, mediating switches between these two networks to facilitate responses to salient stimuli (Uddin 2015). Structural differences related to adversity have generally not been observed in this network. Below, we review existing evidence for associations of adversity with salience network function and connectivity in tasks involving processing of motivationally salient stimuli. Figure 3 and Supplemental Table 3 summarize associations of adversity exposures with activation of the insula (Figure 3a) and dACC (Figure 3b).
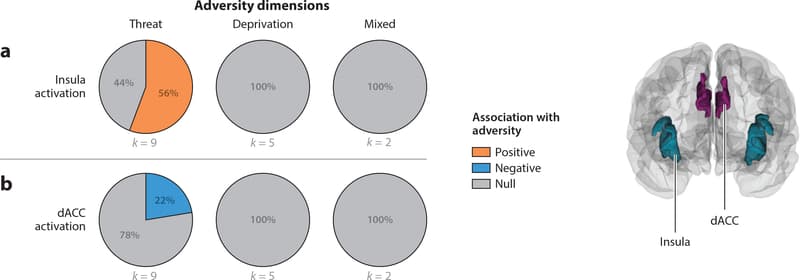
Figure 3: Pie charts represent the proportion of studies that find positive (orange), negative (blue), or null (gray) associations between each adversity type and each measure of neural structure, function, or connectivity. Studies of (a) insula and (b) dorsal anterior cingulate cortex (dACC) activation contrasted activation to negative emotional stimuli compared to neutral emotional stimuli.
Threat
Differences in salience network function have been reported in seven of nine studies (77.8%) in children exposed to trauma, with divergent functional patterns across different nodes of the network. Increased anterior insula activation to threat cues among children exposed to violence was reported in five studies (55.6%) (Cisler et al. 2019, Ganzel et al. 2013, Marusak et al. 2015a, McCrory et al. 2011, McLaughlin et al. 2015) and reduced dACC response to threat cues in two others (Weissman et al. 2019; J.L. Jenness, M. Peverill, A.B. Miller, C. Heleniak, M.M. Robertson et al., manuscript under review). Two studies of threat-related processing reported no differences in salience network regions beyond the amygdala (McCrory et al. 2013, Suzuki et al. 2014).
A similarly divergent pattern of rs-fc of the insula and dACC with the amygdala was observed among children exposed to violence, who had greater rs-fc of the amygdala with insula and lower rs-fc of the amygdala with dACC than children never exposed to violence (Thomason et al. 2015). A final two studies reported other patterns, including reduced insula and dACC activation to trust violations (Lenow et al. 2014) and reduced insula-vmPFC connectivity to fearful versus happy faces (Hart et al. 2018) in trauma-exposed youths.
Deprivation
Functional activation and connectivity differences to threat-related stimuli have not been associated with deprivation in any of the five studies on this topic.
Mixed Exposures
Adversity-related associations with salience network activation have been inconsistent in children with mixed adversity exposures. One study reported greater anterior insula activation in maltreated children with increasing levels of risk in a risk-taking paradigm (Hoffman et al. 2018a). Two others reported lower insula (Puetz et al. 2016) and insula and dACC (Puetz et al. 2014) activity to rejection words and social exclusion, respectively, among maltreated youth. Neither insula nor dACC reactivity to threat cues was related to mixed adversity exposure in two studies (Crozier et al. 2014, Herringa et al. 2016).
A final study reported greater functional connectivity of amygdala and dACC during autobiographical recall of negative versus positive memories in maltreated children (McCrory et al. 2017b).
Summary
Differences in the function and connectivity of salience network regions have been observed consistently in children exposed to trauma, but not deprivation or mixed exposures, during threat processing.
Hippocampal Circuits and Memory
The hippocampus is a subcortical region centrally involved in associative learning and episodic memory (Davachi 2006). The hippocampus binds information across sensory domains through long-term potentiation, a form of plasticity that strengthens connections between neurons that fire together and underlies many forms of learning and memory (Bliss & Collingridge 1993). Connectivity between the hippocampus and PFC underlies memory consolidation and retrieval (Euston et al. 2012). The hippocampus also plays a major role in regulating the hypothalamic- pituitary-adrenal axis, including terminating the response after a stressor has occurred (Jacobson & Sapolsky 1991). In animal models, high levels of cortisol have toxic effects on hippocampal neurons, leading to reductions in dendritic branching and neuronal loss (Brunson et al. 2001, Ivy et al. 2010). For this reason, hippocampal volume has frequently been studied in relation to childhood adversity. Figure 4 and Supplemental Table 4 provide details on all studies examining adversity-related differences in hippocampal volume, function, and connectivity.
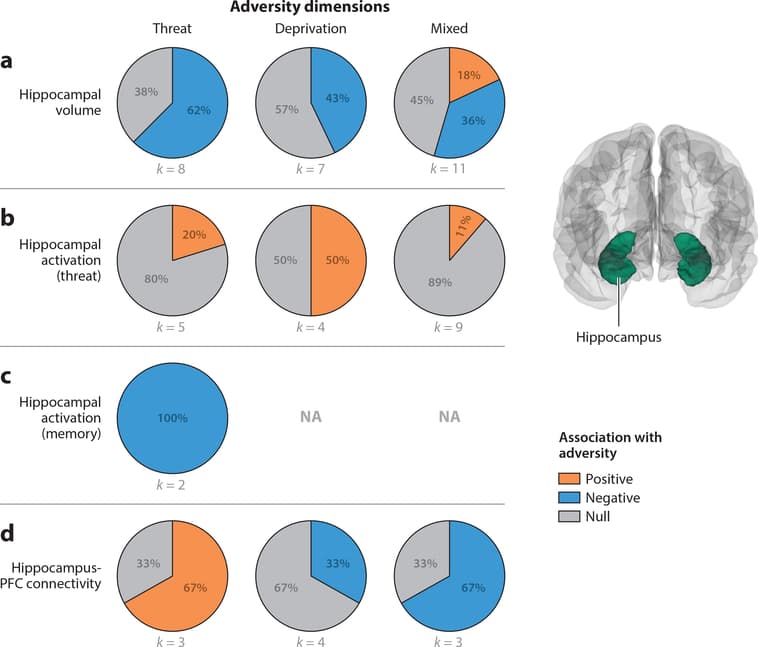
Figure 4: Pie charts represent the proportion of studies that find positive (orange), negative (blue), or null (gray) associations between each adversity type and (a) hippocampal volume, (b) hippocampal activation in response to threat cues, (c) hippocampal activation during memory tasks, and (d) hippocampus-prefrontal cortex (PFC) connectivity. In panel c, hippocampal activation data are not available (NA) for deprivation and mixed exposures. Studies of hippocampus-PFC connectivity summarized in panel d include those using either fractional anisotropy of the cingulum or seed-based resting-state functional connectivity of the hippocampus.
Hippocampal Volume
Figure 4a summarizes associations of threat, deprivation, and mixed exposures with hippocampal volume.
Threat Reductions in hippocampal volume as a function of threat exposure have been found in five (Brooks et al. 2014, Hanson et al. 2015b, McLaughlin et al. 2016, Saxbe et al. 2018; D.G. Weissman, H.K. Lambert, A.M. Rodman, M. Peverill, M.A. Sheridan & K.A. McLaughlin, manuscript under review) of eight studies (62.5%), with no differences in three others (Edmiston et al. 2010, Gold et al. 2016, Lim et al. 2018).
Deprivation Differences in hippocampal volume have not been observed consistently in children exposed to deprivation, with three of seven studies (42.9%) reporting reduced hippocampal volume following deprivation—one in previously institutionalized children (Hodel et al. 2015) and two in relation to physical and emotional neglect (Brooks et al. 2014, Edmiston et al. 2010), and four observing no differences in previously institutionalized children (Hanson et al. 2015b, Mehta et al. 2009, Sheridan et al. 2012, Tottenham et al. 2010).
Mixed exposures Reductions in hippocampal volume in children exposed to adversity have been found in 4 of 12 studies (33.3%) in samples with mixed adversity experiences (Barch et al. 2019, Dahmen et al. 2018, Rao et al. 2010, Redlich et al. 2018); an additional study reported reductions in volume of the parahippocampal gyrus (Dalvie et al. 2014). In contrast, 2 studies reported increased hippocampal volume (Morey et al. 2016, Whittle et al. 2013), and 4 observed no adversity-related differences (De Brito et al. 2013, Kelly et al. 2015, Korgaonkar et al. 2013, Liao et al. 2013).
Summary Reductions in hippocampal volume have been reported most consistently for children exposed to threat-related adversity, inconsistently for those exposed to deprivation, and rarely in samples with mixed exposures.
Hippocampal Activation Figure 4b summarizes associations of adversity exposures with hippocampal activation to threat-related relative to neutral cues and Figure 4c with hippocampal activation during memory tasks.
Threat Differences in hippocampal activation in response to threat cues have generally not been observed, with one of five studies (20%) examining threat processing reporting increased hippocampal activation to fearful relative to neutral faces in children exposed to trauma (Ganzel et al. 2013).Reduced hippocampal recruitment during memory encoding has been observed in children exposed to violence in both existing studies (100%), which involved encoding faces paired with other stimuli (Lambert et al. 2017b, 2019).
Deprivation Hippocampal recruitment in children exposed to deprivation has been examined only in relation to threat processing. Two of four samples (50%) found increased hippocampal activation, one to fearful and angry relative to neutral faces (Maheu et al. 2010) and one to threat relative to safety cues during aversive learning (Silvers et al. 2016b).
Mixed exposures Existing evidence on hippocampal activation in samples with mixed adversity exposures is limited. Enhanced hippocampal activation in response to fearful relative to scrambled faces was found in one of nine studies (11.1%) of threat processing (Crozier et al. 2014). In contrast, increased hippocampal activation during recall of negative relative to positive autobiographical memories (McCrory et al. 2017b) and during reward processing in relation to expected value (Gerin et al. 2017) has been documented in mixed samples.
Summary No consistent patterns have been observed with regard to hippocampal activation in response to threat cues for any type of adversity. In contrast, consistent reductions in hippocampal activation have been found in the small number of studies examining memory tasks specifically among children exposed to threat.
Hippocampus-Prefrontal Cortex Connectivity
Figure 4d summarizes associations of threat, deprivation, and mixed exposures with patterns of hippocampus-PFC structural and functional connectivity.
Threat Few studies have examined hippocampal connectivity in children exposed to trauma, but findings generally suggest greater hippocampus-PFC connectivity in this group. One study observed greater task-based functional connectivity with ventrolateral PFC and reduced connectivity with dorsolateral PFC (dlPFC) during memory encoding (Lambert et al. 2017b). Another observed greater rs-fc of the hippocampus with insula and lateral temporal cortex in adolescents exposed to violence (Saxbe et al. 2018). Finally, higher FA in the cingulum in youth exposed to trauma was reported in a large study of nearly 1,400 youths (Gur et al. 2019), with no trauma- related differences in another small study (Park et al. 2016).
Deprivation One study observed greater functional connectivity of the hippocampus with dmPFC, vmPFC, and dlPFC in previously institutionalized children to threat relative to safety cues during aversive learning (Silvers et al. 2016b). Altered structural connectivity of the hippocampus and PFC has been found inconsistently, with two of six studies (33.3%) reporting reduced FA in the cingulum in children exposed to deprivation (Bick et al. 2015, Kumar et al. 2013).
Mixed exposures Reduced FA in the cingulum has been observed in one of two studies (50%) (Huang et al. 2012) and reduced rs-fc of the hippocampus and sgACC in one study of children with mixed adversity exposures (Herringa et al. 2013).
Summary Few consistent patterns have emerged with regard to hippocampal-PFC connectivity in children exposed to adversity.
Frontoparietal Network and Cognitive Control
One of the neural networks argued to be particularly influenced by experiences of deprivation is the frontoparietal network (Sheridan & McLaughlin 2014), which is involved in working memory and cognitive control (Dosenbach et al. 2007). This network encompasses multiple regions within dlPFC, dmPFC, and superior parietal cortex (Power et al. 2011, Yeo et al. 2011). Here, we focus on studies examining the structure of these cortical regions, including superior frontal and middle frontal gyri, frontal pole, superior parietal lobule, and intraparietal sulcus, and the white matter tracts that connect dlPFC and superior parietal cortex—the superior longitudinal fasciculus (SLF) and inferior fronto-occipital (IFO) fasciculus (Martino et al. 2010, Wang et al. 2016). Given that the dimensional model of adversity argues that disruptions in cognitive control over neutral stimuli will occur primarily following deprivation, whereas difficulties with cognitive control over affectively salient stimuli should emerge in children exposed to both threat and deprivation (Lambert et al. 2017a), we separately review working memory and cognitive control fMRI tasks that examine neutral stimuli (e.g., shapes) from those that have an affective component (e.g., emotional faces). These findings are summarized in Figure 5 and Supplemental Table 5.
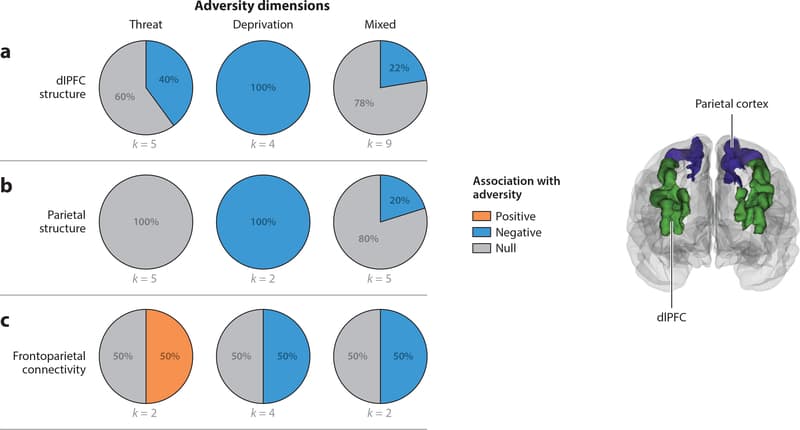
Figure 5: Pie charts represent the proportion of studies that find positive (orange), negative (blue), or null (gray) associations between each adversity type and (a) dorsolateral prefrontal cortex (dlPFC) structure, (b) parietal structure, and (c) frontoparietal connectivity. Studies of dlPFC and parietal structure include studies that used measures of cortical thickness, volume, or surface area. Studies of frontoparietal connectivity summarized in panel c include studies using either structural connectivity of the superior longitudinal fasciculus or the inferior fronto-occipital fasciculus or resting-state functional connectivity.
Frontoparietal Structure
Associations of threat, deprivation, and mixed exposures with structure of dlPFC and superior parietal regions within the frontoparietal network are summarized in Figure 5a,,b,b, respectively.
Threat Two of five studies (40%) have reported reductions in dlPFC volume among children exposed to trauma (Edmiston et al. 2010, Hanson et al. 2010); three have not observed trauma-related differences (Butler et al. 2018, Gold et al. 2016, Lim et al. 2018). No studies (0%) that examined superior parietal cortex structure observed trauma-related differences.
Deprivation Differences in dlPFC structure have been observed consistently in children exposed to deprivation, with all four studies (100%) finding reductions in cortical volume or thickness in this region (Edmiston et al. 2010, Herzberg et al. 2018, Hodel et al. 2015, McLaughlin et al. 2014b). Of the two studies that also reported on superior parietal cortex structure, both (100%) found reductions in thickness (McLaughlin et al. 2014b) or volume (Edmiston et al. 2010) among children who experienced deprivation.
Mixed exposures Reductions in dlPFC volume and thickness have not been observed consistently in samples with mixed adversity exposures, with only two of nine studies (22%) observing such differences (Kelly et al. 2013, 2016) and seven reporting null results (Brooks et al. 2014, Dalvie et al. 2014, De Brito et al. 2013, Korgaonkar et al. 2013, Liao et al. 2013, Luby et al. 2017, Redlich et al. 2018). One of five studies (20%) found lower superior parietal cortex volume among maltreated children (Kelly et al. 2015).
Summary Differences in the structure of the frontoparietal network have been consistently observed in children exposed to deprivation but not among children exposed to threat or with mixed exposures.
Frontoparietal Activation During Cognitive Control
In this section, we review the small number of studies examining adversity-related differences in recruitment in the frontoparietal network during working memory and cognitive control tasks that do not involve emotional stimuli.
Threat Three studies of frontoparietal recruitment during cognitive control tasks in children exposed to trauma have produced mixed findings, with one reporting greater recruitment of dACC and dlPFC during unsuccessful inhibition (Lim et al. 2015), another reporting reduced recruitment of dlPFC during sustained attention (Lim et al. 2016), and a final observing no differences in frontoparietal recruitment during working memory (Sheridan et al. 2017).
Deprivation Only one study has examined neural activation during a cognitive control task in children exposed to deprivation, and greater dACC and insula activation during correct inhibition trials following deprivation was reported (Mueller et al. 2010).
Mixed exposures Two studies have examined neural recruitment during a cognitive control task; one found reduced dACC activation during incorrect relative to correct inhibition trials in children exposed to adversity (Fava et al. 2019) and the other found no adversity-related differences (Jankowski et al. 2017).
Summary Existing studies of frontoparietal network activation during cognitive control tasks are highly mixed for children exposed to threat and in samples with mixed exposures. Too few studies have been conducted in samples with deprivation to draw conclusions.
Frontoparietal Activation During Cognitive Control of Emotion
Here, we focus on tasks involving working memory or inhibition of emotional stimuli (typically faces); effortful forms of emotion regulation (e.g., cognitive reappraisal), which recruits frontoparietal circuits in the service of modulating amygdala activity (Buhle et al. 2014); and studies of rs-fc differences of amygdala with frontoparietal regions.
Threat Three studies have examined neural activation during cognitive control of emotion in children exposed to violence. All observed differences in frontoparietal recruitment, although the specific tasks and pattern of findings varied. Greater activation in dlPFC during inhibition of emotional stimuli (Marusak et al. 2015c) and greater dlPFC, dACC, and frontal pole recruitment during cognitive reappraisal (McLaughlin et al. 2015) have been reported in children exposed to violence. In contrast, reduced dlPFC and intraparietal sulcus activation during encoding in children exposed to violence was observed during working memory for emotional faces (Jenness et al. 2018).
Deprivation In the only study examining cognitive control of emotion in children exposed to deprivation, previously institutionalized children exhibited reduced dlPFC recruitment during inhibition of emotional faces (Tottenham et al. 2010).
Mixed exposures In samples of mixed adversity exposures, reduced dACC activity was observed during correct inhibition of responses to emotional faces in children exposed to adversity relative to controls (Bruce et al. 2013). In addition, higher rs-fc connectivity of the amygdala with dlPFC was observed among children exposed to maltreatment (Herringa et al. 2013).
Summary Children exposed to threat exhibit greater recruitment of frontoparietal regions during cognitive control of emotion, whereas reduced frontoparietal activation has been observed in children exposed to deprivation and in mixed exposure samples. However, these conclusions are preliminary, given the small number of studies on this topic.
Frontoparietal Connectivity This section reviews adversity-related differences in task-based functional connectivity and rs-fc of the frontoparietal network along with structural integrity of the SLF and IFO. Figure 5c summarizes the associations of threat, deprivation, and mixed exposures with these frontoparietal connectivity measures.
Threat Frontoparietal connectivity findings are mixed in children exposed to trauma. One study reported lower functional connectivity in this network during sustained attention in children exposed to abuse (Hart et al. 2017). One of two studies examining frontoparietal structural connectivity observed greater FA in the SLF in children exposed to trauma in a large sample (Gur et al. 2019); another reported no trauma-related differences in a much smaller sample (Park et al. 2016).
Deprivation Two of four studies (50%) examining structural connectivity following deprivation reported reductions in FA in frontoparietal tracts: one in the SLF (Govindan et al. 2010) and one in the IFO (Hanson et al. 2013). Another two studies found no deprivation-related differences (Bick et al. 2015, Kumar et al. 2013).
Mixed exposures One of two studies (50%) reported reduced FA in the SLF in children exposed to adversity (Huang et al. 2012), and the other found no adversity-related differences (Korgaonkar etal.2013).
Summary Findings on frontoparietal connectivity are highly mixed, with no clear patterns across adversity types.
Frontostriatal Circuits and Reward Processing
Dopaminergic circuits in the mesolimbic pathway—encompassing projections from the midbrain to the striatum, and the mesocortical system—involving projections from the striatum to the PFC play a role in organizing behaviors in the approach and consumption of rewards, reward learning, and the experience of positive emotions during the anticipation and receipt of rewards (Berridge & Kringelbach 2008, Berridge et al. 2009). Structural connections in the mesocortical system include the anterior corona radiata, external capsule, and anterior limb of the internal capsule, each of which includes tracts linking the striatum and PFC (Schmahmann et al. 2008). Differences in striatal volume have not typically been observed in children exposed to adversity. Here, we review differences in frontostriatal recruitment and connectivity. These findings are summarized in Figure 6 and Supplemental Table 6.
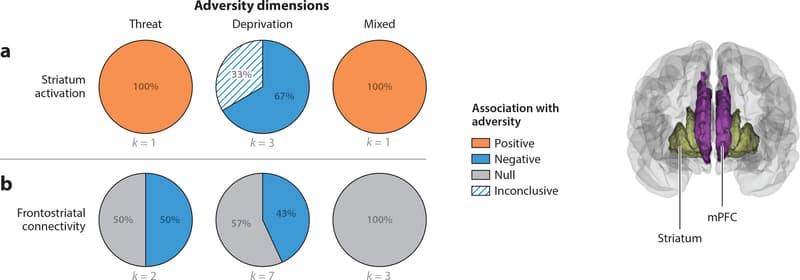
Figure 6: Pie charts represent the proportion of studies that find positive (orange), negative (blue), or null (gray) associations between each adversity type and (a) striatum activation and (b) frontostriatal connectivity. Striatum activation refers to studies that contrasted rewarding or positive emotional stimuli with neutral contrasts. For one of three studies of the relation between deprivation and striatum activation, happy faces were contrasted against the implicit baseline of the intertrial interval instead of a neutral stimulus. Studies of frontostriatal connectivity summarized in panel b include studies measuring either fractional anisotropy of one of the frontostriatal white matter tracts or seed-based resting-state functional connectivity of the striatum. Abbreviation: mPFC, medial prefrontal cortex.
Striatal Activation
We review studies examining recruitment of the dorsal and ventral striatum in tasks involving reward processing. These findings are depicted in Figure 6a.
Threat In the one existing study on threat-related adversity and frontostriatal function, adolescents exposed to trauma exhibited heightened neural response in the dorsal and ventral striatum to positive relative to neutral social images (Dennison et al. 2016).
Deprivation Three functional neuroimaging studies have examined deprivation and striatal function, and all have observed reductions in ventral striatum response to rewarding stimuli among children who have experienced deprivation. This includes reduced activation to reward anticipation (Mehta et al. 2010) and to happy faces relative to baseline in adolescents but not children (Goff et al. 2013), as well as less growth in ventral striatum response to reward receipt across adolescence (Hanson et al. 2015a).
Mixed exposures The one fMRI study of reward processing in a sample with mixed maltreatment exposures reported complicated findings. Decreased activity in trials involving approach- related behavior was observed in the dorsal striatum and orbitofrontal cortex as a function of expected value in maltreated children, increased activity in the midcingulate was observed in relation to prediction error, and no differences were found during reward receipt (Gerin et al. 2017).
Summary Alterations in striatal function during reward processing have been reported consistently among children exposed to adversity, with notably different patterns across adversity types. Whereas deprivation has been consistently associated with reduced striatal recruitment during reward processing, heightened striatal activation has been observed in the small number of studies examining children with trauma and mixed exposures.
Frontostriatal Connectivity
Here, we review studies examining frontostriatal rs-fc and structural integrity of the anterior corona radiata, external capsule, and anterior limb of the internal capsule; no existing studies have reported task-based frontostriatal functional connectivity. Figure 6b summarizes these associations.
Threat Findings on frontostriatal connectivity in trauma-exposed samples are mixed, with one study reporting reductions in FA in the external capsule in children exposed to trauma (Dennison et al. 2019) and another finding no trauma-related differences in frontostriatal tracts (Park et al. 2016).
Deprivation Reduced white matter integrity in frontostriatal tracts has been found in three of seven studies (42.9%) of children exposed to deprivation (Behen et al. 2009, Bick et al. 2015, Dennison et al. 2019); no structural differences in these tracts were observed in four studies (Eluvathingal et al. 2006, Govindan et al. 2010, Hanson et al. 2013, Kumar et al. 2013). In contrast, greater frontostriatal rs-fc was observed among previously institutionalized children relative to controls (Fareri et al. 2017).
Mixed exposures None of the three studies of structural connectivity in samples with mixed adversity exposures observed differences in frontostriatal tracts (Huang et al. 2012, Korgaonkar et al. 2013, Puetz et al. 2017).
Summary Adversity-related differences in frontostriatal connectivity are inconsistent, with no clear patterns in existing studies.
Brain Regions Requiring Further Study
Adversity-related differences have been observed fairly consistently in several other brain regions that we do not review quantitatively. These include the ventrolateral PFC/inferior frontal gyrus (Barch et al. 2018, Luby et al. 2017); ventral visual stream regions (McLaughlin et al. 2014b) and splenium of the corpus callosum (Sheridan et al. 2012, Teicher et al. 2004); and the cerebellum (Bauer et al. 2009). Greater details on adversity-related differences in each of these domains are provided in the Supplemental Text.
Summary
Threat and Deprivation The dimensional model of adversity argues that threat-related exposures should have the strongest influence on amygdala-mPFC and salience network structure and function, whereas deprivation should have stronger associations with the frontoparietal network; both forms of adversity are thought to influence neural networks involved in the cognitive control of emotion. These predictions were largely supported in this systematic review. A majority of studies examining children exposed to violence observed reductions in amygdala volume, increased amygdala reactivity to threat cues, and greater threat-related activation in the anterior insula, a key node of the salience network. These patterns were not consistently observed in children exposed to deprivation. These neural differences are consistent with a long history of behavioral studies demonstrating enhanced threat processing in children exposed to violence (reviewed in McLaughlin & Lambert 2017) and likely reflect an adaptation to growing up in a dangerous environment. Reductions in hippocampal volume were also most consistently observed in children exposed to threat-related adversity.
In contrast, all studies of children exposed to deprivation revealed reduced volume and thickness of the dlPFC and superior parietal cortex, whereas a minority of studies observed this pattern in children exposed to threat. Relatively few studies have examined nonemotional cognitive control tasks in children exposed to adversity, making it difficult to draw firm conclusions in this domain. However, the structural findings are broadly consistent with numerous studies documenting difficulties with cognitive control among children exposed to deprivation (Bos et al. 2009, Pollak et al. 2010). Consistent adversity-related differences emerged in tasks requiring cognitive control over emotional stimuli, but with different patterns for threat and deprivation. Trauma-exposed children consistently exhibited heightened activation in frontoparietal regions when modulating emotional responses, potentially reflecting the need for greater effort or cognitive resources to modulate intense emotional responses; in contrast, children exposed to deprivation and with mixed exposures exhibited reduced activation in these regions during cognitive control of emotion.
Differences in striatal function during reward processing have been observed in children exposed to threat and deprivation, although in different directions—with children exposed to deprivation exhibiting blunted recruitment during reward-related processing and children exposed to threat exhibiting enhanced striatal activation. Few studies have examined this circuit in children exposed to adversity, however, pointing to a need for greater research in this area.
Across all networks reviewed, the findings from samples with mixed adversity exposures were highly inconsistent, with few discernable patterns. This likely reflects the heterogeneity in adversity experiences in these samples and highlights the importance of more careful assessment and modeling of adversity in future studies. Existing evidence is thus broadly consistent with the predictions of the dimensional model of adversity, despite the fact that most studies were not designed to test these predictions directly and few included controls for co-occurring adversity types.
Accelerated Development The central hypothesis of the stress acceleration model is that caregiver deprivation and other forms of early-life stress will accelerate the maturation of the amygdala-mPFC circuit (Callaghan & Tottenham 2016a,b). Existing evidence for this prediction varies across different measures of amygdala-mPFC connectivity. The strongest support is from studies of task-based functional connectivity during threat processing, with half observing a pattern consistent with accelerated development, reflecting stronger negative amygdala-mPFC functional coupling. However, whether such a pattern truly reflects more mature development remains an open question. This assumption is based on an influential study (Gee et al. 2013b), but this age-related pattern has not been replicated in larger studies, including in a recent sample of over 750 children (Zhang et al. 2019). To evaluate the stress acceleration model, greater clarity regarding the typical developmental trajectory of amygdala-mPFC connectivity is required, and patterns of task-related functional connectivity may be too variable across tasks to serve as a reliable metric. Evidence from other metrics of amygdala-mPFC connectivity is mixed in supporting a stress acceleration pattern. Most resting-state studies actually suggest a delayed rather than accelerated pattern of maturation in children exposed to adversity (i.e., reduced amygdala-mPFC rs-fc), and patterns of structural development in the uncinate fasciculus are inconsistent, although in the largest study examining this tract, the pattern observed was consistent with accelerated development in trauma-exposed youths (Gur et al. 2019).
Although findings on amygdala-mPFC connectivity are mixed, evidence for accelerated development following adversity has emerged more consistently for other metrics of neurodevelopment. Cortical thickness and the structural integrity of white matter tracts each exhibit consistent developmental patterns that can be used to evaluate acceleration or delay in children exposed to adversity. Cortical thickness decreases linearly from early childhood to adulthood throughout the cortex (LeWinn et al. 2017, Vijayakumar et al. 2016, Wierenga et al. 2014), whereas the structural integrity of most white matter tracts in the brain increases monotonically from early childhood through early adulthood (Lebel & Beaulieu 2011, Lebel et al. 2012). Reduced cortical thickness and increased white matter integrity are thus two potential markers of accelerated development. Lower cortical thickness among children exposed to adversity relative to age-matched controls was observed in multiple brain networks in our review. These patterns of cortical thickness can be interpreted as reflecting accelerated development among children exposed to both threat and deprivation, albeit in different neural networks. Moreover, a recent study with a very large sample observed greater white matter integrity in children exposed to trauma across tracts spanning multiple networks, including the uncinate fasciculus, cingulum, and SLF (Gur et al. 2019). That same study used machine learning to estimate adulthood based on brain structure and function and found that children exposed to trauma were more likely to be misclassified as adults, suggesting a global pattern of accelerated neurodevelopment. This global pattern of acceleration of neural development in trauma-exposed children is consistent with substantial and long-standing evidence that children exposed to trauma exhibit earlier pubertal maturation than their peers (Mendle et al. 2011, Sumner et al. 2019). In a recent meta-analysis, acceleration in pubertal development as well as cellular aging was observed in children exposed to threat but not in children exposed to deprivation (Colich et al. 2019). Greater research is needed to determine whether acceleration in neural development occurs globally across the brain or only in specific networks and whether these patterns differ as a function of adversity type.
Future Directions
Larger Samples and Longitudinal Data
Existing research on childhood adversity and neurodevelopment is plagued by small, heterogeneous samples and cross-sectional designs. This represents a significant barrier to progress but reflects the inherent logistical and clinical challenges in recruiting large samples of children with exposure to adversity. It is unfeasible for any one lab to recruit and follow a sufficiently large sample of adversity-exposed children to provide adequate power to evaluate the most pressing questions in this area. Investigating distinctions in neurodevelopment across adversity types requires sufficiently large samples of children with exposure to various forms of threat and deprivation; evaluating whether adversity accelerates neural development at minimum requires children with and without adversity exposure across a wide age range and ideally would involve longitudinal tracking of neurodevelopment across time. Overcoming these challenges is unlikely to be feasible for any single investigator or lab. Instead, a critical next step for the field is to develop strategies to pool data across labs; such an approach is the only viable solution to the problems of heterogeneity and lack of statistical power.
To that end, Moriah Thomason, Ryan Herringa, and I are creating a resource for doing so: the Neurodevelopment, Early Adversity, and Resilience (NEAR) Consortium. Our goal is to provide a repository of neuroimaging and clinical data on as many samples of children with adversity exposure as possible. This will allow data to be pooled across much larger groups of children and, ideally, will stimulate progress in understanding the neurodevelopmental consequences of early-life adversity. Participation in the consortium will be open to any investigator with neuroimaging data on children exposed to adversity.
Distinguishing Adversity Types
Adversity experiences frequently co-occur (McLaughlin et al. 2012). Most existing studies combine children with distinct adversity types together for analysis or use cumulative risk scores (Evans et al. 2013) to predict neural outcomes. Such an approach makes it impossible to evaluate whether different forms of adversity have unique neurodevelopmental consequences. As the results of the systematic review reveal, no consistent neural patterns emerged in studies using this type of approach for classifying adversity. To identify specificity, multiple dimensions of adversity must be measured and entered simultaneously into the same statistical model (McLaughlin & Sheridan 2016). Controlling for co-occurring exposures is essential to isolate associations that are unique to different adversity types, if they in fact exist. Otherwise, the likelihood of spurious associations is high, given the co-occurrence of adversity experiences. This co-occurrence creates challenges for interpreting the results of studies that measure only a single type of adversity but is not sufficiently great to introduce problems of multicollinearity when trying to tease apart distinct associations between particular experiences and neurodevelopmental outcomes (McLaughlin et al. 2012). Nonetheless, this approach has rarely been taken in existing work, even in studies that measure multiple adversity dimensions. The goal is not to recruit children with only a single type of adversity exposure but to more carefully evaluate the degree to which different dimensions of environmental experience have overlapping or distinct associations with neurodevelopmental outcomes. Advances in data-driven approaches, including machine learning (Drysdale et al. 2017) and network analysis (Sheridan et al. in press) methods designed to reveal underlying structure in complex, multidimensional data, are likely to stimulate progress in revealing the underlying structure of associations of different adversity types with neurodevelopmental outcomes.
Timing of Exposure
Variability in developmental plasticity as a function of the timing of exposure to environmental experiences is well established and could contribute to the variability in findings across studies included in this review. Sensitive periods reflect developmentally specific periods of heightened neural plasticity, when certain types of environmental inputs are particularly likely to produce reorganization in neural circuits and lasting changes in behavior (Hensch 2005). Sensitive periods in human development have been identified in sensory domains as well as for language, face processing, and attachment (Johnson 2005, Smyke et al. 2010, Werker & Hensch 2015). A fundamental unanswered question, however, is whether the neurodevelopmental consequences of adversity vary as a function of exposure timing. Sensitive period plasticity is an experience-expectant developmental process (Greenough et al. 1987). For children exposed to deprivation, an absence of expected environmental inputs should produce the largest and most lasting changes in neural circuits that process the particular input that is lacking when deprivation occurs during a sensitive period. Remarkably little research has examined this question in relation to MRI-based measures of neural development. Exposure to threat does not reflect an environmental substrate for which a sensitive period is likely to exist (i.e., a developmental period when the brain expects to experience threat). However, trauma could possibly alter the timing of sensitive periods, influencing when they open, close, or both. This could occur as a result of an acceleration in neurodevelopment or by influencing neurobiological factors such as BDNF that serve as triggers for sensitive periods (Bennett & Lagopoulos 2014). To date, empirical research on these questions is limited by the challenges in accurately measuring timing of exposure to adversity and lack of knowledge about the precise timing of sensitive periods for social, emotional, and cognitive development. Advances in neuroimaging tools to measure sensitive period plasticity are needed for progress to be made on these questions.
Expanding Neurodevelopmental Models of Adversity
Empirical work on adversity and neurodevelopment has outpaced the development of theoretical models. Most empirical studies have focused on a relatively limited number of neural processes involving responses to salient negative and positive cues and in tasks that require cognitive control, and numerous findings uncovered in this systematic review are difficult to explain in the context of existing theories. For example, adversity-related differences in the structure and function of visual networks and ventrolateral PFC are not clearly explained by existing models. Although adversity- related differences in the structure and function of multiple regions in the default mode network have been observed, existing theories do not describe how adversity exposure might influence the development of this network. Moreover, associations of adversity with function in large-scale cortical networks and interactions across networks have only recently been examined (Hoffman et al. 2018b, Iadipaolo et al. 2018), and theoretical predictions on how adversity may shape these types of interactions are lacking.
Expanding existing models to encompass additional dimensions of adversity experience is also a critical future direction. Current models focus largely on dimensions of threat and deprivation, although other dimensions of environmental experience such as loss and unpredictability are likely to shape neurodevelopment. Although caregiver deprivation among children who are orphaned or neglected has been studied frequently, the neurodevelopmental consequences of loss of an attachment figure—due to parental death or other forms of forced separation—are currently unknown. Unpredictability is thought to be a primary driver of developmental plasticity and life history strategies that children learn through contingencies in early development (Belsky et al. 2012, Frankenhuis et al. 2013). Rodent models of early-life adversity have also demonstrated that maternal unpredictability and environmental variability can have lasting effects on neurodevelopment (Baram et al. 2012). Extending these models to human neurodevelopment is an important goal for future research, albeit a challenging one, given difficulties in accurately measuring environmental predictability.
Learning Mechanisms
As we recently argued, an important next step for the field will be to shift from salience processing paradigms to those focused on fear and reward-related learning (McLaughlin et al. 2019). Although alterations in emotional learning represent a central mechanism through which early experience influences social and emotional development, there is a remarkable dearth of research examining how childhood adversity influences these forms of learning and their underlying neural substrates. Disruptions in associative learning processes of aversive and appetitive stimuli are well documented in multiple forms of psychopathology (Dunsmoor & Paz 2015, Jovanovic & Ressler 2010, Pizzagalli et al. 2008). Critically, many of the most effective psychosocial interventions for mental disorders directly target these learning mechanisms (Craske et al. 2014, Dimidjian et al. 2006). Although these behavioral techniques form the backbone of evidence-based treatments, they have rarely been studied as potential approaches for preventing the emergence of psychopathology in children exposed to adversity. Greater research into the emotional learning mechanisms linking experiences of adversity with risk for psychopathology has the potential to provide clear targets for early intervention.
Conclusion
An extensive literature on childhood adversity and neurodevelopment has emerged over the past decade. Existing studies are broadly consistent with a dimensional model of adversity, revealing alterations in the structure and function of fronto-amygdala circuits and the salience network in children exposed to threat and frontoparietal network in children exposed to deprivation. Reductions in hippocampal volume are most consistently observed following threat, whereas altered frontostriatal function has been found most frequently following deprivation. Few consistent patterns have been found in studies with mixed adversity samples, likely due to heterogeneity in adversity experiences. Evidence for accelerated development in amygdala-mPFC circuits is inconsistent, although emerging studies suggest that a more global pattern of accelerated neurodevelopment may occur in children exposed to adversity. Despite convergent evidence in these areas, the small and heterogeneous samples and cross-sectional designs that characterize this literature are major barriers to progress in understanding the neurodevelopmental consequences of adversity. Overcoming these barriers will require larger samples and pooled data across labs, more precise assessment and quantification of adversity, and longitudinal studies that track neurodevelopment over time.