Abstract
Purpose A vast literature finds that low self-control is associated with a myriad of antisocial behaviors. Consequently, increasing attention has focused on the causes of low self-control. While criminologists have directed significant attention to studying its social causes, fewer studies have considered its neural bases. Methods We add to this nascent body of research by using data collected on an at-risk sample of adolescents participating in the ongoing Michigan Longitudinal Study. We examine the functioning of prefrontal and limbic regions of the brain during failed inhibitory control, assessed using the go/no-go task and functional magnetic resonance imaging, in relation to low self-control and self-reported delinquency. Results Results indicate that greater activation localized in the anterior cingulate cortex (ACC) during failed inhibitory control is negatively associated with low self-control. Moreover, the association between ACC activity and later delinquency is mediated through low self-control. Conclusions Findings of this study demonstrate the utility of integrating neuroscientific and criminological perspectives on the causes of antisocial behavior. Concluding remarks address the theoretical and policy implications of the findings, as well as directions for future research."
1. Introduction
Since the publication of A General Theory of Crime (Gottfredson & Hirschi, 1990), low self-control has been at the center of criminological research on the causes of delinquency and crime, and a multitude of studies find a positive association between low self-control and various antisocial behaviors (e.g., Burt, Sweeten, & Simons, 2014; Hay & Meldrum, 2015; Vazsonyi, Mikuška, & Kelley, 2017; Wright, Caspi, Moffitt, & Silva, 1999). Given this, scholars have increasingly focused attention on the causes of low self-control. In this regard, criminologists have thoroughly scrutinized its social causes, including parenting practices (e.g., Beaver, Ferguson, & Lynn-Whaley, 2010; Botchkovar, Marshall, Rocque, & Posick, 2015; Hay & Forrest, 2006; Vazsonyi & Huang, 2010; Wright & Beaver, 2005), peer associations (e.g., Burt, Simons, & Simons, 2006; Meldrum & Hay, 2012; Meldrum, Young, & Weerman, 2012), school environments (e.g., Burt et al., 2006; Turner, Piquero, & Pratt, 2005), and neighborhood contexts (e.g., Gibson, Sullivan, Jones, & Piquero, 2010; Jones, 2017; Pratt, Turner, & Piquero, 2004; Teasdale & Silver, 2009).
While much attention has been directed at the social causes of low self-control, other researchers have started to shed light on its neurobiological and neuropsychological underpinnings (e.g., Beaver, DeLisi, Vaughn, & Wright, 2010; Beaver, Wright, & DeLisi, 2007; Cauffman, Steinberg, & Piquero, 2005; DeLisi, 2014; Jackson & Beaver, 2013; Ratchford & Beaver, 2009). This work parallels research taking place within the neurosciences highlighting the importance of a concept closely related to self-control—inhibitory control—for understanding patterns of antisocial behavior (e.g., Feil, Sheppard, Fitzgerald, Yücel, Lubman, & Bradshaw, 2010; Luijten, Machielsen, Veltman, Hester, Haan, & Franken, 2014). Taken together, these lines of research call attention to a neglected aspect of Gottfredson and Hirschi’s (1990)theory by providing evidence for a neurobiological substrate to self-control. To date, however, criminologists have generally examined neural influences on self-control by employing data derived from neuropsychological tests (e.g., Early Screening Inventory; Peabody Picture Vocabulary Test; Tower of London) rather than more proximate measurements of neural activity (but see Yancey, Venables, Hicks, & Patrick, 2013). Thus, additional research based on the measurement of brain activity via neuroimaging techniques (e.g., functional magnetic resonance imaging [fMRI]) can augment the existing criminological literature and further inform ongoing discussion regarding the neural underpinnings of self-control.
In a similar manner, the field of criminology has generally approached the study of the neurobiological bases of delinquency and crime by relying on indirect indicators of neural activity (e.g., Cauffman et al., 2005; Moffitt, Lynam, & Silva, 1994). And, while the relevance of research based on the use of neuroimaging data for the study of criminal behavior has been discussed within criminological journals (e.g., Bufkin & Luttrell, 2005), studies based on the use of neuroimaging data are virtually absent from the criminological literature. This, despite the fact that meta-analytic research assessing the association between structural and functional brain abnormalities and antisocial behavior has already been produced in other disciplines (see Yang & Raine, 2009). Given these facts, coupled with the increased interest in the neurobiological underpinnings of low self-control – a well established cause of delinquency and crime – it would seem important for criminologists to seek out collaborations with other scholars, particularly neuroscientists, to develop a more comprehensive understanding of the neural underpinnings of antisocial behavior.
Accordingly, the current study utilizes fMRI and survey data to examine two issues. First, we investigate whether activity localized in regions of the brain known to be associated with failed inhibitory control, including the insula, dorsolateral prefrontal cortex (dlPFC), and anterior cingulate cortex (ACC), is prospectively associated with variation in self-control during early adolescence. Second, we assess whether low self-control mediates the association between brain activity and later delinquent behavior. In the following section, we discuss theory and research on the causes of low self-control, including the growing discourse concerning its neurobiological underpinnings. Following this, research on failed inhibitory control and error monitoring, and the brain regions associated with failed inhibitory control and antisocial behavior, is discussed. After addressing the goals of the current study and presenting our hypotheses, we describe the prospective data used and present the results of our analyses.
2. Literature Review
2.1 Theory and research on the causes of low self-control
Gottfredson and Hirschi’s (1990) theory of low self-control, articulated in A General Theory of Crime, proposes that individual-level delinquency and crime can be explained by a lack of self-control. In articulating the concept of low self-control, they state that people who are low in self-control will tend to “pursue short-term gratification without consideration of the long-term consequences of their acts” (1990:177). Further, they state that individuals who are low in self-control will “tend to be impulsive, insensitive, physical (as opposed to mental), risk-taking, short-sighted, and nonverbal, and they will tend therefore to engage in criminal and analogous acts” (1990:90). In support of this claim, a large body of research finds that various indicators of low self-control are associated with a wide range of antisocial and deviant behaviors (for meta-analytic reviews see Pratt & Cullen, 2000; Vazsonyi et al., 2017; see also deRidder, Lensvelt-Mulders, Finkenauer, Stok, & Baumeister, 2012). In addition, research finds that low self-control is associated with a variety of negative life outcomes, including criminal victimization, poor health, and financial difficulties (e.g., Hay and Meldrum, 2015; Moffitt et al., 2011; Pratt, Turanovic, Fox, & Wright, 2014).
This evidence calls attention to the need to determine the causes of low self-control. In this regard Gottfredson and Hirschi (1990) place socialization, particularly parental socialization, at the center of the development of self-control. Specifically, they emphasize the importance of parental monitoring of the child’s behavior and the use of effective disciplinary practices when a child misbehaves (1990:97). To the extent that a child is not effectively socialized, deficits in self-control are theorized to be the result. Prompted by this argument, a large number of studies have examined whether parenting practices account for variation in self-control, with many studies providing evidence supporting Gottfredson and Hirschi’s claims (e.g., Botchkovar et al., 2015; Cullen, Unnever, Wright, & Beaver, 2008; Finkenauer, Engels, & Baumeister, 2005; Burt, Simons, & Simons, 2006; Hay, 2001; Hay & Forrest, 2006; Meldrum, Young, & Lehmann, 2015; Perrone, Sullivan, Pratt, & Margaryan, 2004; Vazsonyi & Huang, 2010).
Yet, other studies find that parental socialization has a modest effect on the development of self-control (e.g., Vera & Moon, 2013), particularly once child-driven effects and genetic effects are taken into account (Beaver, Ferguson, & Lynn-Whaley, 2010; Cecil, Barker, Jaffee, & Viding, 2012; Wright & Beaver, 2005; Wright, Beaver, DeLisi, & Vaughn, 2008). Furthermore, studies which have found evidence supporting the link between parental socialization and self-control indicate that parental socialization accounts for a small portion of the variation in child and adolescent self-control (see for example Botchkovar et al., 2015; Perrone et al., 2004). These facts have led researchers to examine additional sources of socialization for instilling self-control, including the school context (e.g., Burt et al., 2006; Turner et al., 2005), peer groups (e.g., Burt et al., 2006; Meldrum & Hay, 2012; Meldrum et al., 2012), and neighborhood environments (e.g., Gibson et al., 2010; Pratt et al., 2004; Teasdale & Silver, 2009). Many of these studies indicate that such sources incrementally add to our understanding of the causes of low self-control above and beyond parenting practices.
While most criminologists have sought to uncover the varied social causes of low self-control, others have started investigating its neural, biological, and genetic underpinnings. This represents a significant departure from the view proffered by Gottfredson and Hirschi (1990) that low self-control is a consequence of failed socialization. Specifically, Gottfredson and Hirschi (1990) are silent with regard to the role of the brain in explaining low self-control and generally reject the role of biological and genetic factors (1990:61–63), going so far as to state that genetic contributions to explaining antisocial behavior (and presumably low self-control) are “near zero” (1990:60). In contrast to this position, a growing number of studies support the contention that a neurobiological substrate also contributes to the variation in low self-control. First, research indicates that self-control is heritable (e.g., Beaver, Eagle Schutt, Boutwell, Ratchford, Roberts, & Barnes, 2009; Boisvert, Wright, Knopik, & Vaske, 2012; Connolly & Beaver, 2014). Second, a number of studies find that early-in-life factors such as birth complications (e.g., Beaver & Wright, 2005) and fetal exposure to neuroteratogens during pregnancy (e.g., Meldrum & Barnes, 2016; Minnes, Min, Short, Wu, Lang, Yoon, & Singer, 2014; Turner, Livecchi, Beaver, & Booth, 2011) are detrimental to the development of self-control.
Most pertinent to our current focus, a growing number of criminologists have directed attention to the potential importance of brain functioning in accounting for between-person differences in self-control (e.g., Beaver et al., 2007; DeLisi, 2014; Jackson & Beaver, 2013). Beaver and colleagues (2007) in particular contend that self-control should be viewed as an executive function of the frontal lobes of the brain (see also Barkley, 1997). The host of executive functions carried out by the brain include many things which overlap with Gottfredson and Hirschi’s (1990) view of self-control, including planning, decision-making, sustaining attention, and inhibiting inappropriate or impulsive behavior (e.g., Moffitt, 1990; Ishikawa & Raine, 2003). To test the relationship between brain functioning and self-control, criminological studies have primarily made use of data derived from neuropsychological tests. Supporting the view that there is a neural basis to self-control, multiple studies report a positive association between neuropsychological deficits and low self-control (e.g., Cauffman et al., 2005; Jackson & Beaver, 2013; Ratchford & Beaver, 2009). Related strands of research also provide evidence that neuropsychological deficits are associated with broad measures of antisocial behavior (e.g., Beaver et al., 2012; Jackson, 2017; Jackson & Beaver, 2016; Jackson & Newsome, 2016; Vaske, Newsome, & Boisvert, 2013).
It is important to point out, however, that such studies rely on the indirect measurement of brain functioning. Thus, an important step in moving this area of research forward is to investigate the association between brain functioning and self-control when utilizing more direct measures of brain activity. In this regard, recent studies report that more direct indicators of brain activity account for between-person differences in self-control. For example, Yancey et al. (2013) reported that smaller amplitude of the P300 brain response assessed using electroencephalogram (EEG) was associated with greater trait disinhibition. More recently, Lee and Telzer (2016), using fMRI, reported that negative network coupling between the right fronto-parietal and limbic resting state networks of the brain was associated with greater self-control, as measured using the Brief Self-Control Scale (Tangney, Baumeister, & Boone, 2004). In addition, they found that self-control mediated the association between network coupling and later substance use.
While discourse on the neurobiological bases of self-control and antisocial behavior has only recently started to gain traction within the field of criminology, other disciplines already take for granted the important role of brain functioning in accounting for antisocial, violent, and criminal behavior (Yang & Raine, 2009). In particular, a large body of research has focused on the concept of inhibitory control and the extent to which inhibitory control during neuroimaging task performance procedures explains variance in antisocial behavior. It is to this literature that we now direct our attention.
2.2 Failed inhibitory control, neural activity, and antisocial behavior
Being able to inhibit impulses and suppress irrelevant stimuli from the environment is a key executive function of the brain that develops gradually from childhood to adulthood (Rubia, Russell, Overmeyer, Brammer, Bullmore, Sharma, et al., 2001). More specifically, inhibitory control, which is the ability to regulate prepotent attentional or behavioral responses, and error processing, the identification and correction of differences between an intended and an executed response to prevent future mistakes, are two fundamental components of cognitive control (Luijten et al., 2014) that have links to aggression, deviance, problematic substance use, and psychopathology (Aharoni, Vincent, Harenski, Calhoun, Sinnott-Armstrong, Gazzaniga, & Kiehl, 2013; Aron, Robbins, & Poldrack, 2004; Taylor, Stern, & Gehring, 2007). These cognitive processes are frequently examined using EEG and fMRI and are often measured with go/no-go and stop-signal tasks (Logan, Van Zandt, Verbruggen, & Wagenmakers, 2014; Luijten et al., 2014; Rubia et al., 2001). During these tasks, participants are asked to respond quickly to frequent “go” trials (e.g., pressing a button in response to all letters of the alphabet except “X” appearing on a screen) and to inhibit responding on either infrequent “no-go” trials (e.g., to the letter “X”) or infrequent “stop” trials (e.g., when a beep is sounded; Aron et al., 2004). During failed inhibitory control (i.e., pressing the button during a “no-go” or “stop” trial), the brain responds to the error that was made, and this is typically interpreted as “error monitoring” or “performance monitoring.”
The brain regions associated with failed inhibitory control and error monitoring have been well characterized through event-related potential (ERP) and fMRI studies (see Luijten et al., 2014 for a review). These regions include parts of the prefrontal and limbic cortices, primarily the ACC, the dlPFC, and the insula (Bari & Robbins, 2013; Luijten et al., 2014). Across multiple studies, the ACC, a subregion of the medial prefrontal cortex, is consistently activated during go/no-go and stop-signal tasks (Aharoni et al., 2013; Bari & Robbins, 2013; Luijten et al., 2014) and is known to be involved in executive functioning and self-regulation (Posner, Rothbart, Sheese, & Tang, 2007; Stadler, Sterzer, Schmeck, Krebs, Kleinschmidt, & Poustka, 2007). More specifically, the ACC has been associated with conflict monitoring (i.e., the likelihood of making errors), response selection, error processing, and avoidance learning (Aharoni et al., 2013).
In addition, the ACC has been posited to promote motor control by facilitating appropriate responses and inhibiting inappropriate responses in rapidly changing contexts (Paus, Petrides, Evans, & Meyer, 1993). Specifically, one neurobiological model indicates that the ACC may relay error information from the basal ganglia and the inferior frontal cortex to motor areas of the brain (Aharoni et al, 2013). In turn, these motor areas make small adjustments or modify behavioral plans based on error processing information, which feeds back to the basal ganglia and frontal cortex to facilitate learning (Holyrod & Coles, 2002).The insula and dlPFC are also consistently identified by neuroimaging studies as being important for the processing of errors (Taylor et al., 2007). Specifically, the insula has been shown to respond more strongly to aware errors relative to unaware errors (Hester, Foxe, Molholm, Shpaner, & Garavan, 2005; Klein, Endrass, Kathmann, Neumann, von Cramon, & Ullsperger, 2007), consistent with its known role in autonomic and visceral sensory processes (Critchley, 2005; Critchley, Wiens, Rotshtein, Ohman, & Dolan, 2004; Verberne & Owens, 1998). Through its anatomical connections with other error-related regions including the ACC and somatosensory cortex (Augustine, 1996), previous work supports the insula’s role in the adjustment of behavior following consciously perceived errors. The function of the dlPFC in error processing and behavioral adjustment, on the other hand, likely relates to its role in cognitive control. For instance, one study found that increased activity in the dlPFC was associated with greater adjustments in behavior following conflict (Kerns, Cohen, MacDonald, Cho, Stenger, & Carter, 2004). Furthermore, activity in this same area was associated with slower reaction times following an error. This post-error slowing is thought to reflect more careful and deliberate action following the commission of an error (Klein et al., 2007).
One conclusion that can be gleaned from the neurobiological literature on failed inhibitory control and error monitoring is the critical role that these mechanisms have on signaling other brain regions involved in executive control and attention (Gehring, Goss, Coles, Meyer, & Donchin, 1993). Thus, underlying impairments in inhibitory control and error processing that can impede the learning process by which future errors are avoided are directly linked to behavioral regulation (Hall, Bernat, & Patrick, 2007). In this regard, there is ample evidence that deficits in inhibitory control and error monitoring are associated with a variety of maladaptive outcomes. For example, one study demonstrated that error-related activity in the ACC during the go/no-go task prospectively predicted future re-arrest among a group of adult offenders (Aharoni et al., 2013). Similarly, reduced activity of the dorsomedial prefrontal cortex, including the ACC, was evident during failed response inhibition among adults with substance use disorders and behavioral addictions compared to healthy controls (de Ruiter, Oosterlaan, Veltman, van den Brink, & Goudriaan, 2012). A recent meta-analysis also supports the link between reduced activity in the right ACC and left dlPFC and antisocial behavior among youth and adults (Yang & Raine, 2009). Taken as a whole, the research reviewed above demonstrates that measures of brain activity that tap into inhibitory control and error processing hold significant promise in adding to our understanding of deficits in self-control and involvement in delinquent behavior.
3. The Current Study
The primary aim of the current study is to test the hypothesis that greater activity in brain regions associated with failed inhibitory control, as measured during an fMRI task, is negatively associated with low self-control during early adolescence. Stated differently, individuals who exhibit greater error-related activation during failed inhibition should exhibit greater self-control. We view this as an important step in advancing the literature on the neural bases to self-control because, as previously discussed, past research conducted within a criminological framework has largely relied on data collected from neuropsychological tests. The discussion in the prior section, however, makes clear that neuroimaging methodologies like fMRI have much to offer the field of criminology (See also Bufkin & Luttrell, 2005), and their use could aid in developing a more complete understanding of the causes of low self-control. Thus, the current study fills an important gap in the existing literature concerning the etiology of low self-control by utilizing more direct measures of brain activity and correlating them with low self-control using prospective data.
A second goal of the current study is to examine the interrelationships between brain activity during fMRI, low self-control, and self-reported delinquency. Specifically, we test the hypothesis that there exists an indirect, negative association between greater brain activity during failed inhibitory control and self-reported delinquency that operates through low self-control. While previous research has established reduced activity in certain regions of the brain is consistently correlated with antisocial behavior, less attention has been devoted to uncovering the mechanism(s) through which such associations operate (Yang & Raine, 2009). In this regard, low self-control seems well situated as a candidate given its established association with delinquency and crime (Pratt & Cullen, 2000; Vazsonyi et al., 2017) and prior research linking failed inhibitory control to antisocial behavior. As we will describe, the data we use are ideal for testing our two hypotheses, as the primary variables of interest (i.e., brain activity, low self-control, and delinquency) are each drawn from different sources and, furthermore, each of these variables are temporally separated in time.In addition to these two goals, it is also important to note that the majority of fMRI studies examining antisocial behavior are conducted among samples of adults (see Yang & Raine, 2009). Thus, our focus on the above associations during adolescence is an additional important feature of the current study. In sum, the current study is well situated for making a significant contribution to our understanding of the neurobiological underpinnings of low self-control and delinquent behavior by drawing on a methodology that seldom appears within criminological journals.
4. Method
4.1 Data
Data for this study are culled from the Michigan Longitudinal Study (MLS), an ongoing, prospective, multi-wave study investigating the development of substance use and dependence (see Zucker, Ellis, Fitzgerald, Bingham, & Sanford, 1996). The MLS follows two community samples. The first is a high-risk sample of families comprised of men convicted of drunk driving who met criteria for an alcohol use disorder (AUD), their son, and their son’s biological mother (whose AUD status could vary). The second is a contrast sample of families drawn from the same neighborhoods as the families comprising the high-risk sample. The families in the contrast sample comprised both low-risk families (i.e., parents without a history of substance abuse) as well as moderate-risk families (i.e., AUD-diagnosed men but not necessarily an AUD-related conviction). Approximately three-quarters (76.3%) of the full MLS sample came from either moderate- or high-risk families. Full biological siblings were also included in the MLS if they were within eight years of the male target child.
Participants (N = 85) for the current study came from 67 families whose children participated in the neuroimaging (i.e., fMRI) portion of the MLS. Fifty-one (60.0%) participants had no siblings participate in the study, 28 (32.9%) participants had one sibling participate in the study, and 6 (7.1%) had two siblings participate in the study. Due to recruitment strategies for the larger MLS study (females and non-White families were included in later waves of the study), the majority of the sample for the current study was male (69.4%) and White (65.9%). Participants were excluded if they had any neurological, acute, uncorrected, or chronic medical illness; current Axis I disorder (not including past mood disorder or current or past anxiety, conduct, attention deficit/hyperactivity, or substance use disorder); current or recent (i.e., within 6 months) treatment with centrally active medications; MRI contraindications such as metal implants or claustrophobia; an IQ less than 70; or history of psychosis in first-degree relatives. Readers are referred to publications by Zucker and colleagues for more information on the MLS samples, study procedures, and recruitment strategies (Zucker et al., 1996; Zucker, Fitzgerald, Refior, Puttler, Pallas, & Ellis, 2000).
In addressing our first research question focused on the association between brain activity and low self-control, data for each of the measures we describe below were available for 85 participants. For our second research question concerned with the indirect association between brain activity and later delinquency operating through low self-control, delinquency data was available for 43 of the 85 participants. This is due primarily to the ongoing nature of the MLS, the fact that some of the adolescent participants have not yet reached the age at which delinquency was assessed for the current study, and the thorough data entry and data cleaning processes that occurs after each participant assessment.1 While these sample sizes are small relative to delinquency studies based solely on the use of survey data, as is commonplace within the field of criminology, neuroimaging studies are typically based on sample sizes that are comparable to, and often times smaller than, those in the current study (see Yang & Raine, 2009). Still, we recognize statistical power and generalizability are two important issues relevant to the current study, and we revisit these issues later in the paper.
4.2 Procedure
Parents and children in the MLS completed assessments following initial recruitment (Wave 1, ages 3 to 5) with subsequent assessments occurring every 3 years (e.g., Wave 2, ages 6 to 8). The current study focuses on assessments collected in late childhood (i.e., fMRI and self-control) and adolescence (self-reported delinquency). Written informed consent from parents and assent from study adolescents were obtained. The Institutional Review Board at the university where the MLS was conducted approved all research protocols.
4.3 Survey Measures
4.3.1 Low Self-Control
For this study, low self-control was assessed, on average, when participants were 12.55 years old (SD = 1.72) using items from the caregiver report of the Achenbach System of Empirically-Based Assessment (Child Behavior Checklist, CBCL; Achenbach, 1991). The CBCL has been used extensively and has been shown to have good reliability and validity. Parents (91% mothers) rated a variety of items using a 3-point scale (0 = not true to 2 = very true or often true). The measure for low self-control used here was derived by taking the sum of 16 of the items contained within the CBCL based on previous work on low self-control (e.g., Hay & Forrest, 2006; Meldrum, Petkovsek, Boutwell, & Young, 2017; Meldrum, Young, Hay, & Flexon, 2012). Example items included: “My child cannot concentrate or cannot pay attention for long,” “My child is impulsive or acts without thinking,” and “My child throws temper tantrums or has a hot temper.” The internal consistency for low self-control in the current sample was good (α = 0.86) and the distribution of scores did not exhibit significant skew (skew = 1.4, kurtosis = 2.5).
4.3.2 Delinquency
Delinquency was self-reported, on average, when participants were 15.54 years old (SD = 1.13) using items drawn from the Antisocial Behavior Checklist-Adolescent version ([ASB-R, Adol] Zucker & Noll, 1980; Zucker, 2005).2 Adolescents were asked to rate how often they had engaged in a variety of behaviors in the last three years using a 4-point scale from “never” (0 times in the last three years) to “often” (10 or more times during the last three years). Twenty-four items were selected from the ASB-R, Adol that reflected delinquent behavior. These items cover a range of behaviors including shoplifting, threatening others, fighting with others, drug selling, and weapon carrying. The internal consistency for the 24-item additive measure of delinquency was acceptable (α = 0.79) and the skew for the distribution was not excessive (skew = 2.12, kurtosis = 5.14).3
4.3.3 Control variables
There is evidence to suggest that parent’s AUD is an important risk factor in the development of dispositional characteristics that undermine adjustment and lead to maladaptive behavior in adulthood (Eisenberg, Haugen, Spinrad, Hofer, Chassin, Zhou, et al., 2010). Accordingly, we included family AUD risk status as a dichotomous control variable (0 = low-risk/control group, 1 = moderate- or high-risk). Adolescent’s biological sex (0 = female, 1 = male) and race (0 = non-White, 1 = White) were also included to control for potential differences in brain activation, low self-control, and delinquency. Further, there is some support that general intellectual ability is associated with task performance and fMRI response (Mattay, Goldberg, Fera, Hariri, Tessirore, Egan, et al., 2003), deviant/criminal behavior (Beaver, Schwartz, Nedelec, Connolly, Boutwell, & Barnes, 2013; Moffitt, 1993a), and low self-control (e.g., Meldrum et al., 2017). Given this, we included a full scale intellectual quotient (FSIQ) based on the Wechsler Intelligence Scale for Children (WISC-3; Wechsler, 1991) as an additional covariate.
4.4 fMRI DATA
4.4.1 Stimuli and Task
A go/no-go task (Durston, Thomas, Worden, Yang, & Casey, 2002; Heitzeg, Nigg, Yau, Zucker, & Zubieta, 2010; Hardee, Weiland, Nichols, Welsh, Soules, et al., 2014; Heitzeg, Nigg, Hardee, Soules, Steinberg, Zubieta, & Zucker, 2014; Cope, Hardee, Soules, Burmeister, Zucker, & Heitzeg, 2016) was used to probe failed response inhibition during fMRI scanning. On average, participants were 11.77 (SD = 1.39) years old at the fMRI scan used for the current study.4 Participants viewed a computer screen while lying flat in the MRI scanner and were instructed to respond (i.e., “go”) with a button press to target stimuli (all letters of the alphabet except “X”; proportion = 75%) and to withhold (i.e., “no-go”) the button press to non-target stimuli (the letter “X”; proportion = 25%). Stimulus duration (i.e., the letter appearing on the screen) was 500 ms. The inter-stimulus interval was 3500 ms, during which time participants saw a black screen with a white fixation cross. Responses (i.e., button presses) that occurred within 3000 ms after stimulus onset were recorded.
Stimuli were presented in a rapid mixed-trial event-related design. Each run comprised 49 trials and lasted 3.5 min, and participants completed five runs. For every non-target stimulus, participants could either respond (false alarm; FA) or withhold a response (correct rejection; CR). Similarly, for every target stimulus, participants could either respond (hit) or withhold (miss). Rates of FAs, CRs, hits, and misses were recorded. Reaction times to FAs and hits were also recorded. Interested readers are referred to Appendix A for descriptive statistics on the fMRI task performance measures for the 85 participants.
4.4.2 fMRI Data Acquisition and Data Plan
Descriptions of the manner in which fMRI data is acquired and used to compute measures of brain activity during task performance procedures are quite technical, and so we provide only a brief description herein. Readers interested in additional details regarding the MRI scanner used to acquire the fMRI data, as well the functional images constructed, are referred to Appendix B. Image processing was done using Statistical Parametric Mapping 8 (SPM8, Wellcome Institute of Cognitive Neurology, Oxford, UK). Three regressors (FAs, CRs, and go trials) were convolved with the standard hemodynamic response function, with event duration 4000 ms from stimulus onset. Six realignment parameters and white matter signal intensity were modeled as nuisance variables. For each participant, images that represented the hemodynamic response (i.e., changes in the concentration of deoxygenated hemoglobin in response to neural activity) associated with failed inhibitory control (i.e., FAs vs. implicit baseline) were computed. Implicit baseline was used as the comparison condition as opposed to all target stimuli (i.e., “go” trials) because of the high frequency of targets relative to the other event types (Devito, Meda, Jiantonio, Potenza, Krystal, & Pearlson, 2013).
One-sample t-tests in SPM8 were used to detect activation associated with false alarms (FAs vs. implicit baseline) at the group level. Anatomical masks of five a priori regions of interest (ROIs) based on previous work on error monitoring were created in WFU PickAtlas (Maldjian, Laurienti, Kraft, & Burdette, 2003): bilateral ACC, left insula, right insula, left dlPFC, and right dlPFC (see Figure 1). We then extracted beta values using MarsBaR (Brett, Anton, Valabregue, & Poline, 2002) and imported them into SAS version 9.4 (SAS Institute Inc., 2013) for the analysis.
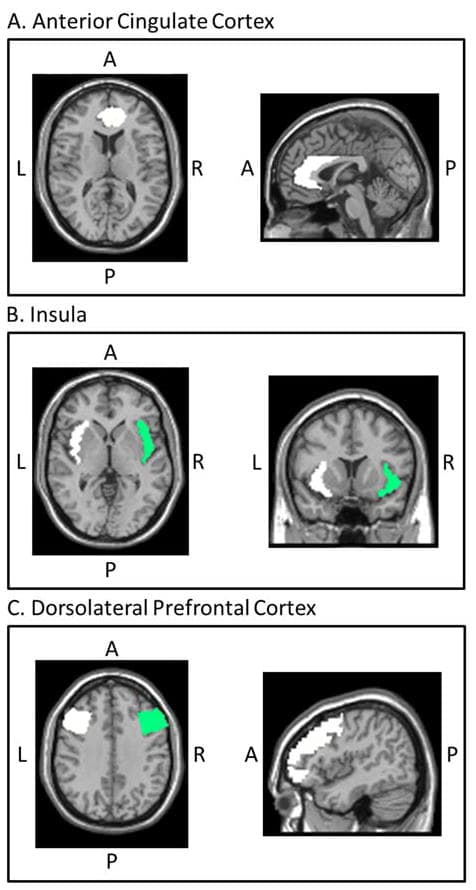
Figure 1: Inhibitory control/error monitoring regions of interest
Anatomical masks of a priori regions of interest (ROIs) based on previous work on error monitoring were created in WFU PickAtlas (Maldjian et al., 2003): bilateral anterior cingulate cortex (A), left and right insula (B), and left and right dorsolateral prefrontal cortex (C). L = left, R = right, A = anterior, P = posterior. Image coordinates in Montreal Neurological Institute space are as follows: anterior cingulate cortex, x = 0, y = 40, z = 12; insula, x = 8, y = 12, z = 0; dorsolateral prefrontal cortex, x = −46, y = 5, z = 36.
5. Results
As a first step, we examined bivariate correlations between activity during failed inhibitory control for the five ROIs described above and the measure of low self-control (after implementing a Bonferroni correction: .05/5 ROIs; p = .01). An examination of these bivariate correlations indicated that only the bilateral measurement of the ACC was significantly associated with low self-control (r = −0.24, p < .01); none of the other four ROIs were significantly associated with low self-control after the Bonferroni correction. Given this, the remainder of our analyses focused exclusively on the measure of ACC activation during failed inhibitory control in relation to low self-control and delinquency.
Table 1 presents the means, standard deviations, and bivariate correlations between ACC activity during failed inhibitory control, low self-control, delinquency, and the control variables. As indicated above, low self-control was negatively associated with greater ACC activation during failed inhibitory control (r = −0.24). Low self-control was also positively associated with later delinquency (r = 0.36). Of additional interest, adolescents from families with AUD risk were lower in self-control relative to adolescents from families with no AUD risk (r = 0.33). Last, at the bivariate level, the association between ACC activation and delinquency was not statistically different from zero. It is important to note, however, that from a statistical perspective a zero-order correlation between the independent variable (i.e., ACC activity) and the dependent variable (i.e., delinquency) is not necessary to establish an indirect effect (see Hayes, 2009).
We next investigated in a multivariate framework whether individual differences in ACC activity prospectively predicted low self-control, net of the control variables. Given that a portion of the sample includes siblings from the same family, they cannot be treated as independent observations because they are likely to share characteristics (e.g., family AUD, family socialization, genetic predispositions) that would make them more similar to each other compared to adolescents from different families (Jenkins, Rasbash, & O’Connor, 2003). Given this possibility, an intercept only model was estimated (random intercept with no predictors) using the mixed procedure in SAS to calculate family clustering effects. Intraclass correlations (ICCs; Raudenbush & Bryk, 2002) were calculated from clustering effects of family for low self-control and delinquency. Results indicated that the amount of variance accounted for by the clustering effect of families on low self-control (ICC = 0.36, p < .05) and delinquency (ICC = 0.83, p < .001) was significant. Accordingly, we estimated multilevel analyses accounting for family clustering.
Table 2 presents the results of the random coefficients (random intercept with adolescent-level predictors) hierarchical linear model accounting for family clustering. Providing support for our first hypothesis, the model indicates that greater ACC activation during failed inhibitory control is negatively associated with lower self-control (b = −0.74, p < .01), net of controls. Put differently, participants who exhibited greater activation in the ACC during failed inhibitory control were rated by parents as having more self-control approximately one year later. In addition, the model indicates that family AUD risk is positively associated with lower self-control (b = 4.00, p <.001). None of the remaining covariates are significantly associated with low self-control. Overall, the predictors in the model accounted for approximately 17% (adjusted R2value) of the variance in low self-control.
The final step of the analysis examined whether an indirect association exists between ACC activity and delinquency via low self-control for participants where delinquency data were available (n = 43). Recall that the measurement of these three key constructs occurred at different points in time (i.e., brain activity first, low self-control second, delinquency third). This prospective design helps establish temporal precedence, a key criterion for establishing mediation (Kraemer, Kiernan, Essex, & Kupfer, 2008). The PROCESS macro for SAS (Model 4; Hayes, 2013) was used to estimate indirect effects. The use of the PROCESS macro in SAS is justified for two reasons: It generates direct and indirect effects, and bootstrapped samples can be requested to derive bias-corrected confidence intervals for indirect effects (Efron & Tibshirani, 1993). Bootstrap confidence bands of mediated effects are viewed as more robust since indirect effects are often non-normally distributed (Preacher, Rucker, & Hayes, 2007). A bootstrap sample of 10,000 was used as recommended (Hayes, 2013).
The results from the PROCESS model are presented in Figure 2. As shown, and consistent with the results presented in Table 1, ACC activation is negatively associated with lower self-control (b = −1.08, p < .01), while family AUD risk is positively associated with lower self-control (b = 3.26, p < .05). The remaining covariates are not associated with self-control. Figure 2 also shows, as would be expected from prior research (Vazsonyi et al., 2017), that lower self-control during early adolescence is prospectively associated with delinquency during middle adolescence (b = 0.30, p < .05). Pertinent to our second hypothesis, Figure 2 shows there is no evidence for a direct effect of ACC activation on later delinquency. There is, however, evidence for a significant indirect (i.e., mediated) effect of ACC activation on later delinquency via low self-control (indirect effect = −0.32, bias-corrected 95% confidence interval = −0.82 – −0.01), with approximately 77% of the total effect of ACC activation on delinquency operating through low self-control.
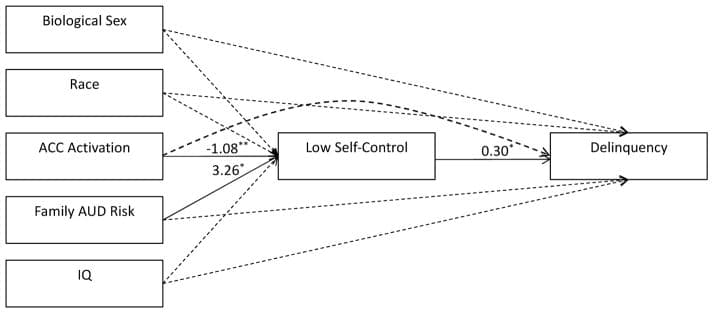
Figure 2: PROCESS model testing indirect effect of ACC activation on delinquency Significant effects are represented by solid lines, while dashed lines represent non-significant effects. ACC = anterior cingulate cortex, AUD = alcohol use disorder, IQ = intelligence quotient. ** p <.01, * p <.05 (two-tailed)
As a robustness check, we ran the same PROCESS model but excluded siblings. Results were largely comparable. That is, there was evidence of an effect of ACC activation on low self-control (b = −1.38, p < .001), family AUD risk was positively associated with low self-control (b = 3.37, p < .05), and low self-control was positively associated with delinquency (b = 0.34, p < .05). Moreover, the indirect effect of ACC activation on delinquency via low self-control remained statistically significant (indirect effect = −0.47, bias-corrected 95% confidence interval −1.32 – −0.04).5
6. Discussion
Inquiry into the causes and consequences of low self-control has become a major focal point of much criminological research (DeLisi, 2011). Prompted by Gottfredson and Hirschi’s (1990) argument that the primary factor that determines one’s level of self-control is parental socialization during childhood, early work on the causes of low self-control focused almost exclusively on testing this hypothesis. With the passage of time, knowledge gained from early studies, and the integration of perspectives on self-control stemming from other disciplines, we now know that the sources of self-control are far more varied than Gottfredson and Hirschi (1990) suggested; social, biological, and genetic factors each appear to play important roles in the development of self-control (Hay & Meldrum, 2015).
Along these lines, prior work within the field of criminology has considered how indirect indicators of neural functioning (i.e., neuropsychological tests) are associated with low self-control and delinquency (e.g., Cauffman et al., 2005; Jackson & Beaver, 2013; Ratchford & Beaver, 2009). Less consideration, however, has been given to the more direct measurement of neural activity (Yancey et al., 2013). We believe this stems in part from the fact that many criminologists lack familiarity with and access to neuroimaging data. The result is that, at present, research into the neurobiological bases of antisocial behavior has largely been conducted with little involvement on the part of criminologists. We believe, however, that transdisciplinary collaborations have utility in advancing this area of research.
In an effort to move the field in this direction, this study examined the potential neurobiological underpinnings of low self-control and delinquent behavior by making use of fMRI data targeting failed inhibitory control collected on a longitudinal sample of high-risk adolescents participating in the ongoing MLS. Three findings emerged which speak to the importance of considering neural activity when seeking to explain low self-control and delinquency. First, of the five brain ROIs considered, greater ACC activity during an fMRI performance task was negatively associated with parental reports of low self-control measured approximately one year later. Second, and consistent with past research (Pratt & Cullen, 2000; Vazsonyi et al., 2017), low self-control during early adolescence was positively associated with delinquency measured approximately three years later during middle adolescence. Third, we found a significant indirect effect of ACC activity on later delinquency mediated through low self-control.
These findings are consistent with and add to the nascent body of research implicating neurobiological factors in the etiology of self-control (Lee & Telzer, 2016; Yancey et al., 2013). Likewise, the finding that ACC activity has an indirect association with later delinquency is also consistent with the well-established finding in the neuroscience literature that ACC functioning is a robust correlate of antisocial and violent behavior among adults (Yang & Raine, 2009). As has been noted by Yang and Raine (2009), however, while many studies have examined the association between neural functioning and antisocial behavior, less attention has focused on investigating the mechanisms through which this association operates. Our findings offer evidence that low self-control serves as one of these mechanisms (see also Lee & Telzer, 2016).
We should remind readers, however, that the ACC was the only tested region significantly correlated with low self-control. In this regard, it is important to note that the five regions tested (i.e., insula, dlPFC, and ACC) were selected for their consistent association with failed inhibitory control during the go/no-go task. Despite a wealth of prior work indicating the importance of the ACC in particular in both failed inhibitory control (in terms of the fMRI task) and behavior regulation, we tested the other regions for completeness and because this is one of the first studies to take a criminological perspective on the associations among brain activity, low self-control, and delinquency using a prospective design. Therefore, it is not necessarily surprising that activity in the other regions failed to reach significance in their association with low self-control when applying a relatively strict Bonferroni correction. It will be important for future work with larger samples to continue to address this question in order to further characterize the importance of various brain regions in the development and expression of low self-control and delinquency.
The above notwithstanding, the findings of our study linking ACC activity to low self-control and later delinquency have important implications for the field of criminology. In particular, they support the call (e.g., Bufkin & Luttrell, 2005) for criminological perspectives on antisocial behavior to give greater consideration to the role of neural functioning. While some perspectives have long emphasized the importance of neurobiological factors in explaining chronic offending over the life course (e.g., Moffitt, 1993b), most criminological theories as originally formulated have little to say about the role of the brain. Therefore, it will be important that future work considers how neural activity might fit into other theoretical frameworks within criminology. For example, recent work indicates that neighborhood disadvantage may impact brain functioning (e.g., Umbach, Raine, Gur, & Portnoy, 2017), a finding which informs social disorganization theory (Shaw & McKay, 1942) and other social structural theories of antisocial behavior. Likewise, other work points to the influence of harsh and physical discipline by parents on neural development (Tomoda, Suzuki, Rabi, Sheu, Polcari, & Teicher, 2009), which is relevant to arguments stemming from general strain theory (Agnew, 1992). Such examples, coupled with the findings of this study as they apply to self-control theory, illustrate the benefits of the integration of neurobiological models into existing theoretical frameworks within the field of criminology to provide a more complete understanding of the etiology of antisocial behavior.
Our findings also hold implications for policy and practice. Primarily, they direct attention to the importance of preventing neural deficits during childhood that could retard the development of self-control and contribute to involvement in later delinquent behavior. Alterations in neural structure and functioning are known to result from such things as food insecurity (Bourre, 2006), head injury (Levin & Hanten, 2005), and exposure to maltreatment (Twardosz & Lutzker, 2010) during childhood. Such findings are relevant to our current focus in light of additional research linking food insecurity, head injury, and maltreatment to deficits in self-control and involvement in antisocial behavior (e.g., Farrer & Hedges, 2011; Jackson & Vaughn, 2017; Jackson, Vaughn, & Salas-Wright, 2017; Kimbro & Denney, 2015; Kort-Butler, Tyler, & Melander, 2011; Schwartz, Connolly, & Brauer, 2017; Shields, Cicchetti, & Ryan, 1994; Vaughn, Salas-Wright, Naeger, Huang, & Piquero, 2016). Given the above strands of research, programming efforts focused on preventing abnormal brain development should be promoted. In this regard, several programs, including the Nurse-Family Partnership program, the Stop Now and Plan (SNAP) program, and the Triple P–Positive Parenting Program, have demonstrated positive results related to brain functioning (Woltering, Granic, Lamm, & Lewis, 2011), cognitive achievement and child behavior (Olds, Kitzman, Cole, Robinson, Sidora, Luckey, et al., 2004), and reductions in child maltreatment (e.g., Prinz et al., 2009). Given these findings, our recommendation is that such efforts be expanded to serve an even larger percentage of the population.
Despite the strengths of this study, including the use of prospective data on a high-risk sample, the use of multiple sources of data to measure key constructs, a focus on a critical period of adolescent development, and the use of fMRI data, there are certain limitations that must be discussed. First, even though the sample size of the current study is comparable to many fMRI studies, it is small relative to most criminological studies, which typically number in the hundreds or thousands. As such, it is possible that different conclusions could emerge with larger samples. Likewise, our focus was on a sample of adolescents that was heavily seeded for elevated risk of antisocial behavior, even though the lower end of our sample’s risk distribution was in the normal range. Thus, our findings may not generalize as well to broader community samples. Second, due to missing data that would have further reduced our sample size, we were unable to consider additional covariates that past research has found to be associated with self-control and delinquent behavior, particularly parenting practices. Given this, future work should assess the influence of brain functioning on self-control when additional factors can be controlled.
In addition to overcoming the limitations noted above, future research should consider whether biological sex and race differences exist in the associations found herein, something we did not consider given the restricted sample size. Future research might also examine how the influence of certain genetic predispositions on low self-control is mediated by neurobiological functioning, in light of work from other disciplines finding that genetic predispositions influence antisocial behavior via neurobiological mediation. Lastly, given emerging links between health and lifestyle-related variables and low self-control (e.g., lack of sleep, poor nutrition, and head injury) which likely operate by altering brain structure and functioning, future work would benefit from a more explicit integration of the neurobiological and health-related literatures into criminological discourse on the causes of low self-control and delinquent behavior.
In conclusion, this study highlights the importance of the role of neurobiological factors in accounting for between-individual differences in self-control and delinquent behavior. Given the near complete absence of neuroimaging studies within criminological journals, we believe there are several fruitful avenues for researchers to consider going forward. The formation of collaborative partnerships between criminologists and those outside of the field with requisite knowledge and access to neuroimaging data, as was done for this study, will be critical in this regard. Doing so would not only foster greater interdisciplinary research, but also aid efforts to develop more comprehensive models of delinquent and criminal behavior.
Highlights - Reduced anterior cingulate cortex (ACC) activity is associated with lower self-control. - Lower self-control is associated with greater delinquency. - Low self-control mediates the association between ACC activity and delinquency. |