Abstract
Adolescence is the transition from childhood to adulthood that begins around the onset of puberty and ends with relative independence from the parent. This developmental period is one when an individual is probably stronger, of higher reasoning capacity, and more resistant to disease than ever before, yet when mortality rates increase by 200%. These untimely deaths are not due to disease but to preventable deaths associated with adolescents putting themselves in harm's way (e.g., accidental fatalities). We present evidence that these alarming health statistics are in part due to diminished self-control—the ability to inhibit inappropriate desires, emotions, and actions in favor of appropriate ones. Findings of adolescent-specific changes in self-control and underlying brain circuitry are considered in terms of how evolutionarily based biological constraints and experiences shape the brain to adapt to the unique intellectual, physical, sexual, and social challenges of adolescence.
Introduction
Over the past decade, the teen brain has received significant attention from the media, due in part to the many seeming contradictions in teen behavior and in part to developments in brain imaging that provide the opportunity to look under the hood of the teen brain (Casey 2013). This is a time when sensation seeking is at an all-time high (Steinberg et al. 2008) yet also a time when anxiety and mood disorders peak (Kessler et al. 2005, Merikangas et al. 2010). So what brain changes take place during adolescence that may explain these seeming inconsistencies in behavior?
Too often in describing the adolescent brain we suggest it has no brakes or steering wheel (Bell & McBride 2010), as if it is defective in some way. However, we don't characterize other formative years of development as defective (Steinberg 2012). When a newborn is unable to talk or walk we do not refer to this inability as a deficit but rather as normal development. Yet the adolescent who makes a bad choice in the heat of the moment among peers is described as having no frontal lobe or as being deviant in some way. Rather than depicting the teen brain and teen behavior as defective, this review portrays a brain that is sculpted by evolutionarily based biological constraints and experiences as it adapts to the unique intellectual, physical, sexual, and social challenges of adolescence.
Defining Adolescence
Adolescence can be defined as the transition from childhood to adulthood that begins around the onset of puberty and ends with relative independence from the parent. Although today financial independence from parents may not be achieved until the offspring is well into the forties or fifties, we refer to independence as when the individual has the capacity to live independently, so by the early twenties for most. Historically, adolescence has been viewed as a period of storm and stress (Hall 1904). Such a characterization seems warranted, with a 200% increase in preventable deaths (accident, suicide, homicide) and a rise in psychopathology during this developmental period (Casey et al. 2014, Paus et al. 2008). However, the mere fact that you are reading this review implies that we are not all doomed to make poor decisions that lead to fatal outcomes during this period. The extreme view of G. Stanley Hall's (1904) inherent storm and stress of adolescence and Margaret Mead's (1928) counter theory emphasizing society and experiences suggest large individual variability in behavior during this developmental phase. Thankfully, developmental psychology has moved beyond simplistic nature-versus-nurture debates, recognizing that behavior reflects interacting environmental and genetic factors that impact the brain's ability to adapt to changing environmental demands. Some environments are expected and some are specific to the individual's experiences. How well we adapt to changing environmental demands of adolescence, with the many physical, sexual, social, and intellectual challenges faced during this period, is thus a function of evolutionarily-based biological constraints and experiential history.
Approaches to Understanding Adolescence
Two important approaches have been used to understand the adolescent brain and behavior: translation and transition (Casey et al. 2010). Each is described below to provide a framework for the developmental studies reviewed in this article.
The first approach involves translational studies across species to understand evolutionarily conserved behaviors. Adolescent behaviors such as novelty seeking, spending more time with peers, and engaging in more fighting with parents are not specific to humans (Spear 2010). Rather, this constellation of age-typical behaviors is present across mammalian species (Spear 2010). Despite the associated risks, these behaviors are thought to serve a number of adaptive functions (Crockett & Pope 1993, Irwin & Millstein 1986, Spear 2010) that include increasing the probability of reproductive success and obtaining additional resources or food. Exploring adult liberties may lead to a greater ability to face and surmount challenges (Csikszentmihalyi & Larson 1987, Daly & Wilson 1987, Meschke & Silbereisen 1997). Because adolescence is an evolutionarily conserved phase of development, we can use animal models to identify and test mechanisms that underlie brain and behavioral changes during adolescence in studies that would not be feasible in humans.
The second approach underscores the importance of understanding the developmental transitions from childhood to adolescence and adolescence to adulthood. Traditionally, developmental stages have been studied in isolation, with psychologists becoming experts in infant psychology, child psychology, or adolescent psychology. Some of our best developmental psychology textbooks today still parse development in these ways. However, how would we know if a process is specific to adolescence without understanding the processes that precede or follow adolescence? Clearly, understanding if some systems develop before others, and how these systems differentially interact across development, is important for understanding any stage of development. It is the cumulative consequences of interacting systems that alter the course of development and give rise to distinct trajectories and functions (Masten & Cicchetti 2010).
Figure 1 illustrates the approach of examining developmental transitions. Adolescent-specific and adolescent-emergent development shows inflections (or deflections) and peaks (or troughs) during the teen years, whereas adolescent-nonspecific changes are those that continue to show a steady increase or decrease from childhood to adulthood (Casey 2013, Somerville et al. 2013). Too often adolescent brain development has been viewed as part of a linear process. This article illustrates how these approaches have identified nonlinear changes in development during adolescence and led to neurobiological accounts of this developmental period.
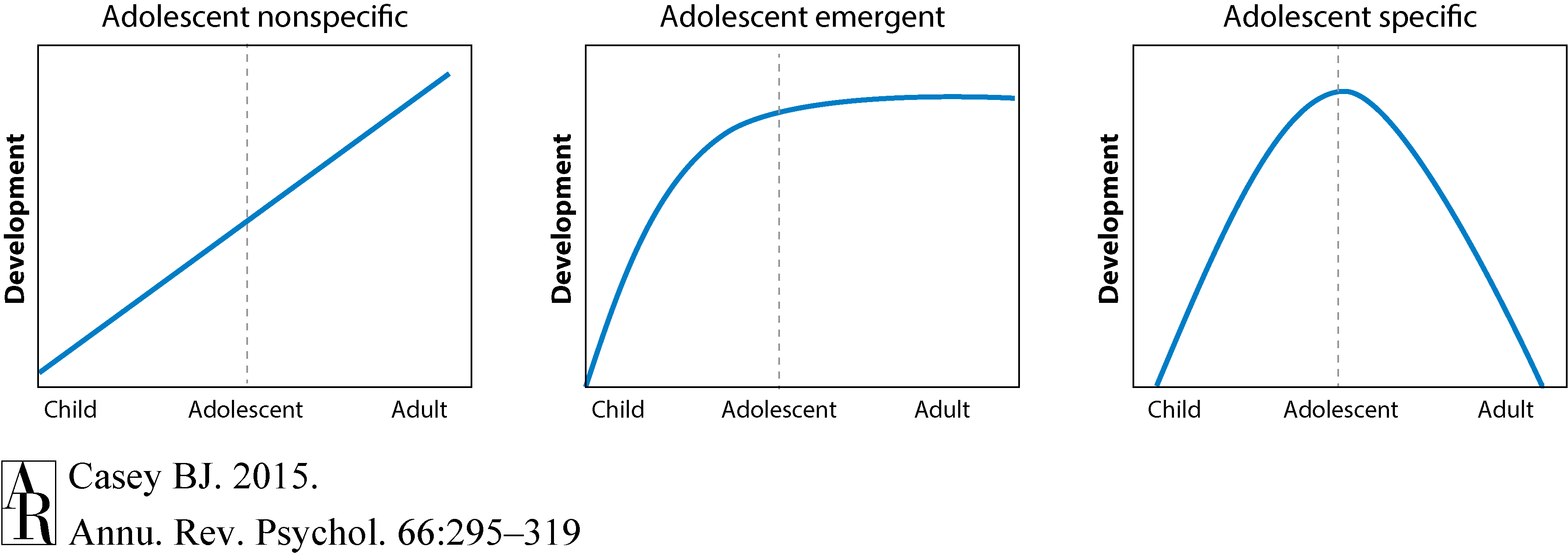
Figure 1 Casey (2013) and Somerville et al. (2013), with permission.]Download Full-ResolutionDownload PPT
Neurobiological Models of Adolescence
A number of neurobiological models have emerged over the years to explain adolescent behavior (Figure 2). One of the most influential of these models is the dual-system model based largely on Metcalf & Mischel's (1999) two-system model of willpower. According to their model, self-control results from a balance between a “cool” and a “hot” system. The cool system is an emotionally neutral, strategic, and flexible cognitive system, whereas the hot system is emotionally driven by fears, desires, and reflexes. A common analogy used to describe these two systems is based on characters of the popular TV series and later movie series Star Trek: the logical Vulcan, Mr. Spock, and the passionate captain of the Starship Enterprise, Kirk, with Spock being the cool system and Kirk being the hot system. Metcalf and Mischel acknowledge that the balance between these two systems varies as a function of the individual, development, and stress.
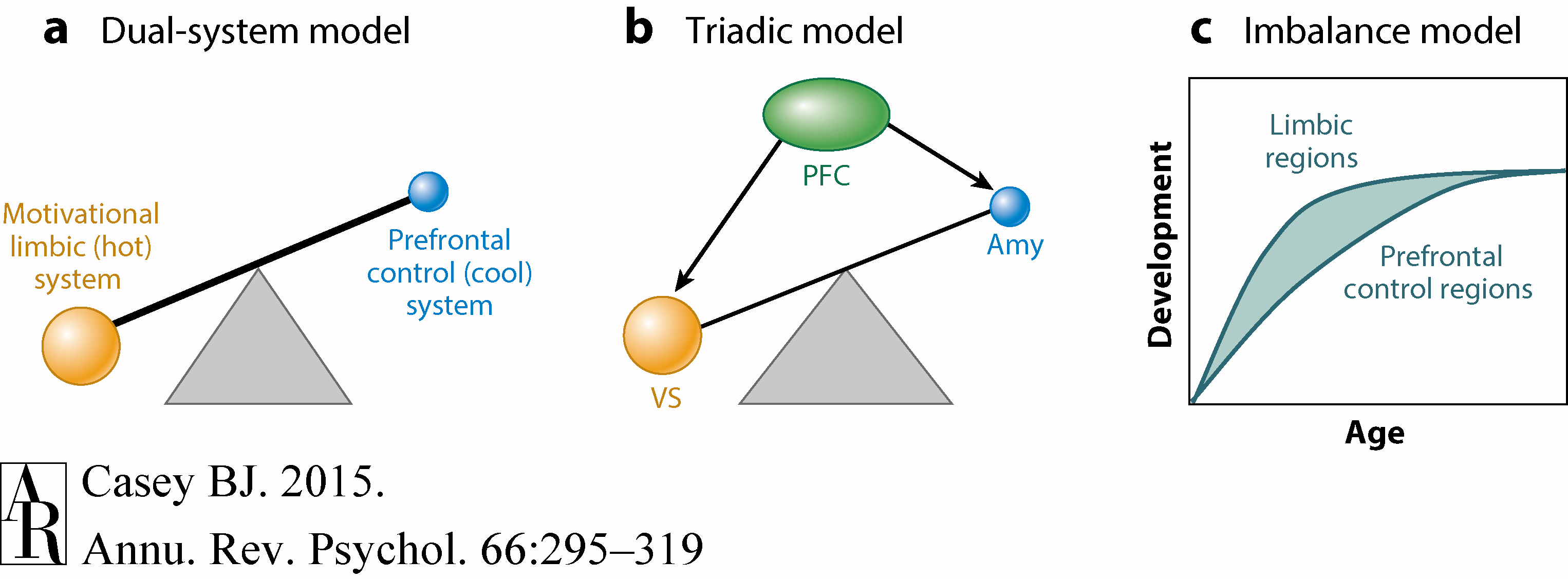
Figure 2
Subsequently, McClure and colleagues (2004) used the dual-system model to heuristically explain brain systems underlying behavior involving immediate versus delayed rewards. Portions of the limbic system correlated with midbrain dopamine were associated with decisions involving immediate rewards, whereas “vulcanized” prefrontal circuitry (Cohen 2005) was associated with decisions to delay rewards. Steinberg et al. (2008) later used this dual-system model to describe differences observed between adolescents and adults in sensation seeking and risky decisions. According to this view, puberty serves to enhance signaling in the limbic or hot system, which in turn is suggested to hijack the more rational prefrontal control system.
In parallel, Ernst and colleagues (2006) proposed a triadic model of motivated behavior to explain differences between adolescents and adults. Specifically, they dissected the limbic, or hot, system into two subsystems: a reward and an avoidance system attributed to the ventral striatum and amygdala, respectively. According to this model, motivated behavior stems from a balance between the reward-driven and harm-avoidant systems, with adolescence being a time when there is a tilt or bias toward the reward-driven system. This bias is proposed to be driven by changes in gonadal hormones resulting in a stronger reward-related system, a weaker harm-avoidant system, or a weak regulatory control system (Ernst et al. 2006).
A third model is referred to as the imbalance model (Casey et al. 2008a). This model is grounded in empirical studies that examine the full developmental transition from childhood through adolescence into adulthood and translation across species (nonhuman primate and rodent). According to this model, regional neurochemical, structural, and functional brain changes within development lead to imbalances within brain circuitry that may explain nonlinear changes in behavior during adolescence.
Evidence of regional brain development comes from human imaging and postmortem studies and nonhuman animal studies. Early evidence for regional brain development was provided by studies of synaptogenesis and synaptic pruning across development (Bourgeois et al. 1994, Huttenlocher & Dabholkar 1997). These studies showed that sensorimotor and subcortical regions reach adult numbers of synapses before association areas such as the prefrontal cortex. Complementary findings of regional brain development come from human imaging studies showing that cortical thickness in sensorimotor cortices peaks in late childhood, before association cortices, which do not peak until early adulthood (Gogtay et al. 2004). Additional evidence from animal studies shows regional changes in availability of neurotrophic factors and neurochemicals. For example, within the dopamine system, a neurotransmitter system implicated in learning and prediction of reward, receptor density peaks during early adolescence in the striatum (Benes et al. 2000, Brenhouse et al. 2008) but not until early adulthood in the prefrontal cortex (Cunningham et al. 2008, Tseng & O'Donnell 2007). Likewise, concentrations of brain-derived neurotrophic factor, which is essential for learning and development, show an earlier peak and subsequent drop in the striatum relative to the cortex (Katoh-Semba et al. 1997).
All three examples above provide evidence for differential development of brain regions within corticosubcortical circuitry that may result in an imbalance or overreliance on one region over another in driving behavior during adolescence. This tension is presumably not observed in childhood because there is a relative lack of maturity across and between regions within the circuit, and in adults, there is a relative maturity of the underlying neurocircuitry. With development and experience, connectivity within brain circuitry is strengthened and provides a mechanism for top-down modulation of the subcortical output that diminishes emotive processes and habitual actions observed more subcortically.
Although all three models provide nice heuristics for conveying why adolescents may engage in certain behaviors, their continued use in framing empirical studies may hinder new developmental discoveries (Helfinstein & Casey 2014, Somerville et al. 2014). For example, new findings have moved the field away from simplistic one-to-one mappings of the ventral striatum and amygdala to reward and avoidant behaviors, toward the recognition of distinct computational roles they each play in learning that influence adaptive action in response to both positive and negative outcomes (Levita et al. 2009, Li et al. 2011, O'Doherty et al. 2003, Roesch et al. 2010). Furthermore, orthogonal or simple dichotomies for hot and cool brain systems fail to appreciate the rich interconnections within and across circuits that may regulate or intensify emotions, desires, and actions. Incorporation of circuit-based changes with development may improve our understanding of why adolescents engage in the behaviors they do and uncover why some circumstances during adolescence compromise self-control more than others.
What Is Self-Control?
Self-Control: A Definition
Self-control may be defined as the ability to suppress inappropriate emotions, desires, and actions in favor of alternative, appropriate ones. One classic example of self-control is the ability to resist temptation of an immediate reward for a later larger reward, known as delay of gratification (Mischel et al. 1988). Mischel's delay-of-gratification task assesses young children's capacity to choose between eating one marshmallow, or a preferred treat, immediately or waiting several minutes, thereby earning the opportunity to have two. Typically, behavior on this task falls along two clusters, with some children eating the treat almost instantaneously or with little delay and others waiting up to 25 minutes to obtain the two treats. This example illustrates how self-control can be detected and measured early in development (Mischel et al. 1989). This article delves into changes in the behavioral and neural correlates of self-control during adolescence and specifically during situations involving desires, emotions, or actions.
Circuitry Underlying Self-Control
First, let's examine the brain circuitry that supports self-control. A simplified illustration of cognitive and motivational circuitry is provided in Figure 3. This figure highlights three key brain areas and their interconnections: the amygdala, prefrontal cortex, and ventral striatum. Each of these regions has been implicated in different computations and functions, and each shares interconnections (Groenewegen & Berendse 1990, Krettek & Price 1977, Wright & Groenewegen 1995). The prefrontal cortex, implicated in reasoning and behavioral regulation, can modulate activity in both the amygdala and ventral striatum to suppress outputs that otherwise lead to emotive responses and actions. The ventral striatum, a region implicated in learning and prediction of rewarding outcomes, also receives inputs from the basolateral amygdala. The amygdala, a region important in associative learning and determination of the emotional significance of cues in the environment, can facilitate striatal activity through direct inputs or suppress striatal activity via indirect inputs through the prefrontal cortex (Stuber et al. 2011). It is the interconnections among these regions that change with development to integrate cognitive and emotional processes and lead to motivated and goal-oriented actions (Ambroggi et al. 2008). Finally, this circuitry is also modulated by dopamine and by hippocampal inputs (Floresco & Maric 2007, Ishikawa & Nakamura 2003), as illustrated in Figure 3, which suggests that behavior may be flexibly modified by salient cues or contexts.
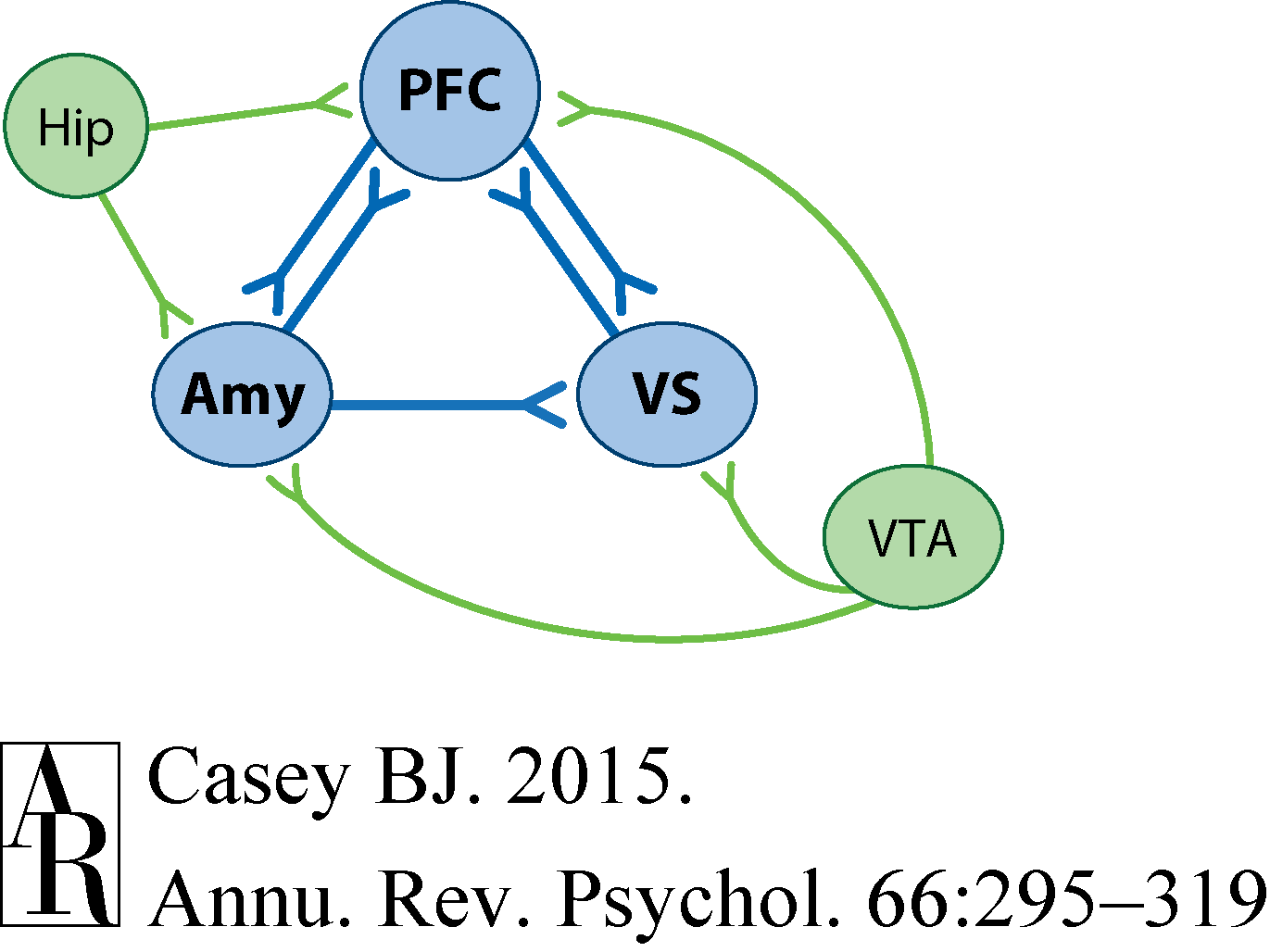
Figure 3
Circuit characterizations of development suggest a number of ways in which the adolescent brain may differ from the child or adult brain. First, protracted development of the prefrontal projections to limbic subcortical structures may lead to less top-down regulation of heightened emotive responses (Dreyfuss et al. 2014, Somerville et al. 2011). Second, less top-down prefrontal modulation of the basolateral amygdala input to the ventral striatum may facilitate motivated action (Stuber et al. 2011). Third, the earlier peak in dopamine receptor density in the striatum (Benes et al. 2000, Brenhouse et al. 2008) relative to the prefrontal cortex (Cunningham et al. 2008, Tseng & O'Donnell 2007) may result in an imbalance or overreliance on subcortical regions in driving behavior.
With this background, we now focus on two areas of the greatest concentration of evidence for adolescent-specific changes in brain and behavior from both human and rodent work. The first involves control of actions in the face of incentives, desires, and peers (i.e., positively valenced cues and contexts), and the second involves control of emotions and actions in the face of potential threat (i.e., negatively valenced cues and contexts). We emphasize the importance of considering the neurocircuitry underlying this behavior rather than regional changes, especially within frontolimbic circuitry that underlies self-control.
Self-Control in the Face of Incentives
Self-control as measured by laboratory cognitive tasks steadily improves from childhood to adulthood. These tasks require participants to suppress attention and actions toward irrelevant information in favor of attention to relevant information and actions to achieve a goal (Casey et al. 2002). This developmental pattern is supported by a wealth of behavioral evidence from psychological tasks including the Simon task, go/nogo task, and attention-switching tasks (Casey et al. 2002). However, the capacity for self-control changes in the context of incentives.
Incentives can alter behavior in at least two ways. When we are rewarded for accurate performance on a given task, we may try harder and perform better than when we are not rewarded. However, our capacity to exert control over our behavior may be compromised when we are required to suppress attention or actions toward cues previously associated with positive outcomes. Perhaps the best illustration of a potential breakdown in self-control when incentives are present is the findings from the delay-of-gratification task in toddlers described previously (Mischel et al. 1989). Mischel and colleagues examined how well a toddler could resist an immediate reward of a marshmallow in favor of a larger reward later (e.g., two marshmallows). Although some children could wait, many could not delay sufficiently long to receive the two marshmallows. Those who could not delay spent much more time focusing on the salient treat rather than not attending to it (Eigsti et al. 2006). Individuals vary in their ability to redirect attention from salient information and delay pleasure even as adults, yet developmental studies suggest windows of development when they may be particularly susceptible to temptations. This ability is a form of self-control because it requires goal-directed behavior in the face of salient, competing inputs and actions. The studies described below indicate that sensitivity to environmental cues, especially socially relevant cues, changes during adolescence and suggest a unique influence of motivation on cognition or self-control during this developmental window.
Appetitive Cues
Several imaging studies have attempted to map the developmental representation of rewards in the brain to explicate how motivationally relevant cues impact behavior (Ernst et al. 2006; Galván et al. 2006, 2013; Geier et al. 2010; van Leijenhorst et al. 2010). One of the first studies to examine neural correlates of reward processing across the full range from childhood to adulthood was completed by Galván and colleagues (2006). Building on prior nonhuman primate studies showing the importance of reinforcement learning and prediction error in dopamine-rich circuitry (Cromwell & Schultz 2003, Fiorillo et al. 2003), investigators varied the magnitudes of monetary reward to discrete stimuli. The ventral striatum and orbitofrontal cortex were shown to be sensitive to cues that predicted the largest reward (Galván et al. 2005, 2006). The response in the ventral striatum to large rewards relative to smaller ones was exaggerated in adolescents compared to both children and adults (Galván et al. 2006). Differential activity in the ventral striatum to monetary rewards in adolescents has been observed across a number of labs and in experimental tasks (Bjork et al. 2008, 2010; Ernst et al. 2006; Galván et al. 2006; Geier et al. 2010; van Leijenhorst et al. 2010). But how does this altered sensitivity to rewards impact self-control?
A number of behavioral studies provide examples of how adolescent behavior is affected in motivational contexts. Using a gambling task in which reward feedback was either provided immediately following a choice or delayed, Figner and colleagues (2009) showed that adolescents made disproportionately more risky gambles to immediate reward feedback compared to adults. A similar gambling task and a delay discounting task (Cauffman et al. 2010, Steinberg et al. 2009), were used to show that this sensitivity to rewards has an adolescent-specific peak around 15 years of age. Together, these studies suggest that motivational cues that signal potential reward are particularly salient because they diminish effective self-control and lead to risky choices in adolescents.
Recent imaging studies have begun to try to elucidate the neural basis for this developmental pattern. Somerville and colleagues (2011) used an impulse control (go/nogo) task together with positive social cues (smiling faces) that over a lifetime come to be associated with positive outcomes. Relative to both children and adults, adolescents showed a diminished capacity to suppress a response toward a rare (unexpected) positive social cue (happy faces). This behavioral pattern was paralleled by an adolescent-specific peak in ventral striatum activity (see Figure 4). In contrast, ventrolateral prefrontal activity showed a linear change with age and was associated with overall accuracy on nogo trials regardless of social cue type (positive or neutral). A functional connectivity analysis revealed stronger local subcortical coupling during adolescence and enhanced prefrontal corticosubcortical coupling in adulthood. Thus, regional changes in circuit connectivity with age were associated with the developmental differences in the behavior.
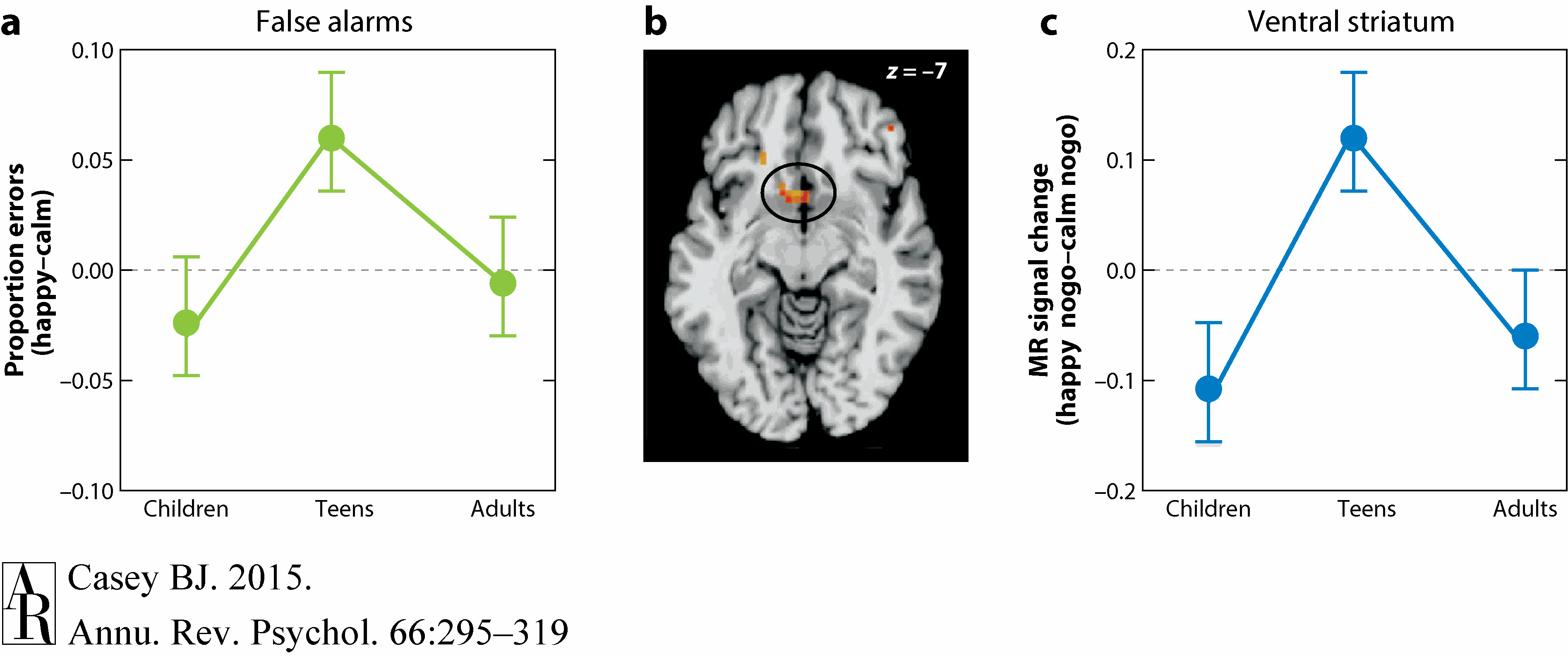
Figure 4 Somerville et al. (2011) with permission.
What is the potential mechanism that explains why adolescents respond more impulsively to positive cues than either children or adults do? Cohen and colleagues (2010) suggest that this adolescent-specific effect may be due to greater positive prediction error (detecting when an unanticipated rare reward occurs). They showed that ventral striatal activity to unpredicted large rewards was greater in adolescents than in children and adults. More recent learning studies suggest that the developmental differences in response to rewards may not be due to changes in learning signals per se but rather to how these signals drive behavior (van den Bos et al. 2009, 2012). Together, these studies suggest that connections between subcortical regions implicated in reward prediction error (ventral striatum) and the prefrontal cortical decision regions change as a function of age, not learning.
Performance-Based Incentives
The previous studies show how cues of potential reward can diminish self-control in adolescents. However, it has long been known that reinforcement can change and improve behavior (Skinner 1938). Given that adolescents appear to have a heightened sensitivity to rewards, do they benefit more than adults from incentives when the reward is dependent upon how well they perform? A clever study used an antisaccade task that required the participant to look in the opposite direction from a cue to measure self-control under rewarding and nonrewarding conditions (Hardin et al. 2009). Participants were informed that they would be financially rewarded for accurate performance on some trials but not on others. The anticipation of a reward facilitated performance on the task for adolescents more than for adults. Geier and colleagues (2010) later showed that this improvement in performance was paralleled by exaggerated activity in the ventral striatum in adolescents relative to adults in preparing and executing the antisaccade. This activity was coupled with increased activity in regions of the prefrontal cortex important for controlling eye movements, suggestive of reward-mediated upregulation of cognitive control regions.
Unfortunately, these studies controlled for neither baseline performance nor the perceived value of reward for the different age groups. If baseline performance were near ceiling for the adults and not for adolescents, then adults would have less capacity for improvement in their performance no matter what the reward. Furthermore, if the rewards used to motivate performance had more perceptual value for the adolescents than the adults, then that too could be a basis for the developmental differences in behavior performance (i.e., certain amounts of money may subjectively feel like more to an adolescent than to an adult).
In an attempt to address these potential confounds, Teslovich et al. (2014) used a perceptual decision-making task for which task difficulty could be individualized. The number of dots moving coherently in one direction on a background of randomly moving dots was varied, and a step function was used to equate performance level across individuals regardless of age. Points rather than money were used as a reward in an attempt to equate the perceived value of rewards, given that even adults use point systems (e.g., points for frequent traveler programs or restaurant reward programs). Large (five points) and small (one point) rewards were mapped to a specific direction of moving dots and were received for correct responses only. Rather than responding impulsively to the direction corresponding to the larger reward, adolescents slowed down, as if letting sufficient evidence accumulate before making a decision to ensure receipt of the five points. This behavioral pattern was paralleled by greater activity of prefrontal and parietal regions implicated in decision making in adolescents relative to adults. These findings suggest that when large rewards are at stake, adolescents are quite capable of not responding impulsively in order to optimize their outcomes.
Together, these studies suggest that sensitivity to motivational cues is enhanced during adolescence and paralleled by changes in ventral striatal activity. This striatal activity appears to differentially modulate the capacity for self-control in cortical control regions in adolescents compared to children or adults. This capacity can be enhanced or impaired, depending on whether suppression of or attention toward these cues is necessary or whether accurate behavior is required to receive a reward. However, motivated behavior rarely occurs in isolation but rather in social settings or with others present. So how do social contexts affect the capacity for self-control?
Social Contexts
Social contexts impact behavior throughout the life span, but perhaps never as much as during adolescence (Blakemore & Mills 2014, Somerville 2013, Steinberg et al. 2008). The mere presence of a peer increases risky decision making in adolescents relative to adults (Chein et al. 2011, Gardner & Steinberg 2005, Weigard et al. 2014) and increases the number of car accidents for adolescent, but not adult, drivers (Chen et al. 2000). Substantial evidence shows that adolescents are more oriented toward and influenced by peers than are either children or adults (Guyer et al. 2009, 2012; Masten et al. 2009; Somerville et al. 2013; Steinberg & Monahan 2007), which is indirectly linked to the dopamine-rich region of the ventral striatum (Chein et al. 2011). Steinberg and colleagues have interpreted these findings as suggesting that peers prime heightened motivational states that then activate the individual, leading to diminished capacity for self-control.
Similar peer effects have been shown in rodents. In a recent study in mice (Logue et al. 2014), alcohol consumption was tested in juveniles and adults while alone or in the presence of cage mates. Increased alcohol consumption was observed among adolescent mice tested in the presence of peers but not in adults in the presence of peers. These findings suggest that the observed peer influence in adolescent risk taking may reflect an evolutionarily conserved process across species.
To explain the influence of peers on adolescent behavior, it has been suggested that peers serve as reinforcers to influence behavior (Chein et al. 2011). Support for this hypothesis comes from a growing literature that shows overlapping neural circuitry for social (praise, gain in reputation, positive affect) and nonsocial (juice, money) rewards (Bhanji & Delgado 2013, Fareri et al. 2012, Izuma et al. 2008, Jones et al. 2011, Lin et al. 2012, Meshi et al. 2013, Rademacher et al. 2010, van den Bos et al. 2007). Jones et al. (2014) developed a social reinforcement learning paradigm to evaluate the degree to which peers serve as reinforcers in promoting and sustaining behavior in adolescents and to test this hypothesis from childhood to adulthood. The investigators manipulated the probability of the participant receiving positive social feedback from three virtual peers, who provided 33%, 66%, or 100% positive feedback. The results from this study showed that different amounts of positive social feedback enhanced learning in children and adults, but all positive social reinforcement from peers equally motivated adolescents, based on quicker response latencies to all three cues. All ages showed elevated medial prefrontal and ventral striatal activity to cues that predicted the most reinforcement. However, adolescents, unlike children and adults, showed increased activity in premotor circuitry when receiving positive social feedback regardless of the expected outcome, suggesting that peer interactions may motivate adolescents toward action.
Together, these findings suggest that adolescence is a time of heightened sensitivity to incentives (money, peer acceptance) and contexts (in the presence of peers), and this heightened sensitivity appears to be supported by changes in dopamine-rich regions of the brain (ventral striatum) that are important for learning and predicting the outcomes of actions. Just as incentives and social contexts influence self-control differently across development, so too do cues of potential threat.
Self-Control in the Face of Threat
It is often assumed that adolescents are fearless and perceive themselves to be invincible or immortal. Yet evidence is building to suggest the contrary. For example, in a large self-report study of over 500 teenagers and adults, Cohn et al. (1995) found that adolescents actually overestimate the risk of fatal outcomes from injury or illness relative to adults. Likewise, adolescents are capable of appreciating the consequences of their actions, yet in the heat of the moment consequences are not taken into account (Casey et al. 2008a), possibly due to a variety of influences including peers, the environmental context, and the emotional state (Gardner & Steinberg 2005, Steinberg 2005). In these emotionally charged situations, environmental cues appear to win over reason. We turn to how emotions, specifically to potential threat in the environment, differentially impact the capacity for self-control across development.
Cues of Potential Danger
Perhaps the most compelling cue for humans of impending danger is the fearful expression of another human. This type of social cue comes to be associated with the potential threat that something bad is about to happen through a lifetime of experiences, although we may be biologically predisposed to view fearful facial expressions as threat related (Öhman & Mineka 2001). The fight-or-flight response to a perceived threat is a physiological reaction (LeDoux 1996). Fearful faces are a reliable predictor of immediate threat in the environment and have been shown to inhibit behavior. This behavioral inhibition is evidenced by a slowing in response times to fearful cues compared to either neutral or happy cues (Hare et al. 2005), but is this response uniform across development?
The capacity to learn about threats in the environment is a highly adaptive skill that is conserved across species. Without this ability we would be unable to detect and avoid danger that could lead to our own demise. When cues of potential threat cease to predict threat (e.g., a snake in the grass versus a snake in a pet store), it is adaptive to suppress the fear response. A growing literature suggests that fear learning develops early. However, the capacity to express and suppress these fear memories changes with development (McCallum et al. 2010; Pattwell et al. 2011, 2012) and depends largely on whether the fear association is specific to a cue or an environmental context.
A number of studies have examined changes in fear circuitry across development to identify changes in how fear is processed. The primary region of focus has been the amygdala and nuclei within this structure. This region is critical for associative learning and detecting the emotional significance of information in the environment (Davis & Whalen 2001, LeDoux 2002). The amygdala receives information about the external world via inputs to the lateral nucleus from the thalamus and maintains fear memories within this nucleus (Maren 2001).
The fear response emerges when a potential threat is detected, and it readies the individual to flee, fight, or even freeze through coordinated physiological responses. The fear response is triggered via projections from the lateral nucleus to the central nucleus. The central nucleus projections to the brain stem, hypothalamus, and autonomic nervous system lead to the behavioral expression of fear. When the potential threat is no longer present, the fear response is quieted or extinguished. Fear extinction occurs via projections from the infralimbic cortex to inhibitory cells in the amygdala that in turn inhibit the output of the central nucleus and suppress the fear response (LeDoux 2002, Phelps et al. 2004). Extinction of a prior fear memory with exposure to new associations does not overwrite the fear memory but rather suppresses it. The persistence of fear memory following extinction learning is evidenced by its reemergence with the passage of time. The hippocampus, which projects both to the prefrontal cortex and amygdala, provides contextual information that influences whether the fear memory or the extinction memory is expressed.
Several groups have examined how fear is differentially represented in the brain in adolescents relative to adults, focusing specifically on the amygdala. Across these studies the magnitude of brain activity to expressions of potential threat (negative facial faces) appears to be higher in adolescents than in adults (Baird et al. 1999, Guyer et al. 2008, Monk et al. 2003). Changes in the brain and behavior in adolescents relative to both adults and children have been examined to determine whether this pattern of brain activity is specific to adolescents (Hare et al. 2008). As illustrated in Figure 5, adolescents showed heightened amygdala activity to fearful face cues relative to children or adults, and this activity was correlated with slower reaction times when fearful cues were detected compared to either neutral or happy cues (Hare et al. 2008). In contrast, activity in the ventromedial prefrontal cortex, the analog to the rodent infralimbic cortex (Phelps et al. 2004), was associated with quicker responses to fearful cues. Furthermore, habituation of the amygdala response was tightly coupled with this ventromedial prefrontal activity, suggesting its importance in downregulating emotional responses.
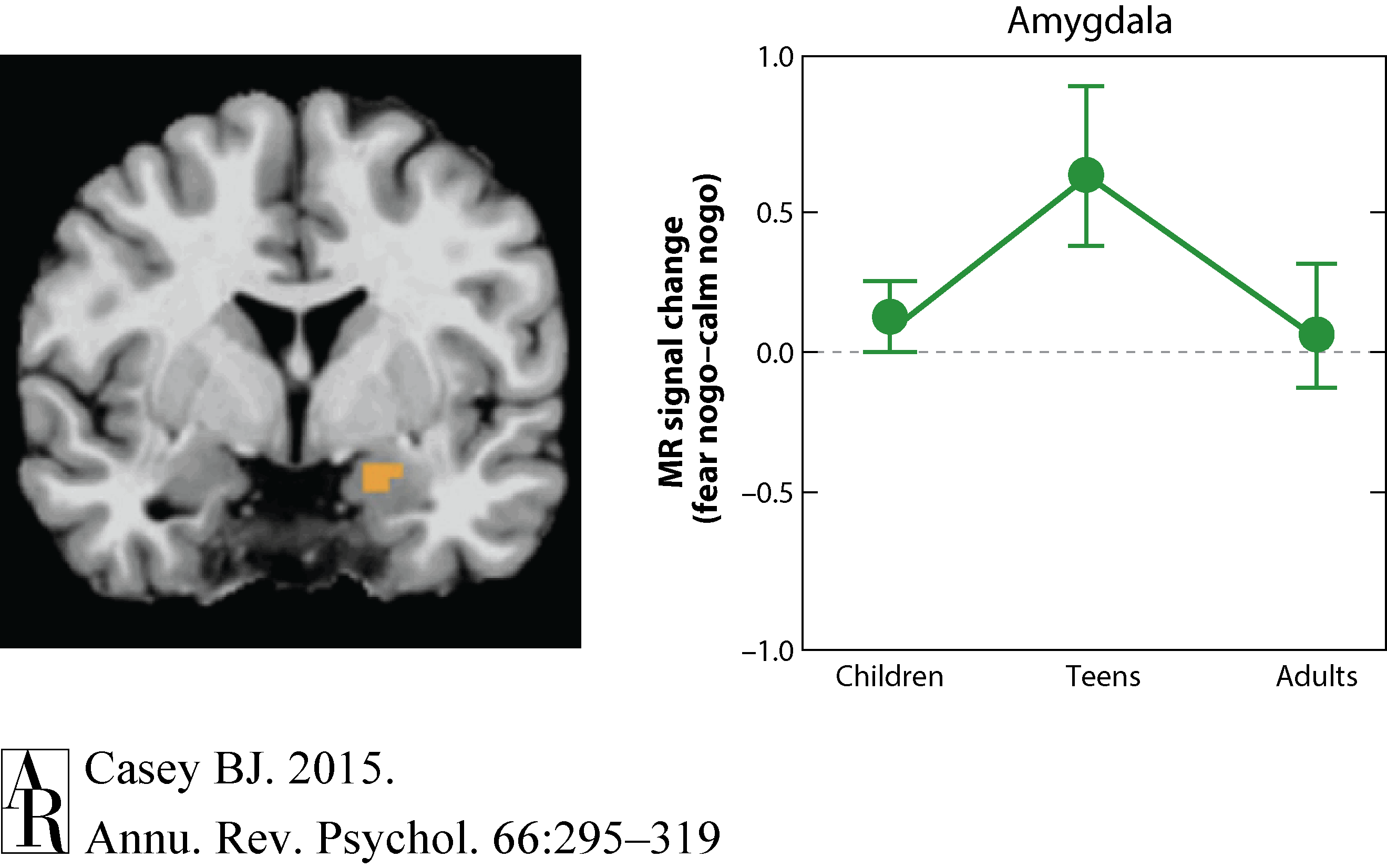
Figure 5 Hare et al. (2008) with permission from Elsevier.
Developmental imaging studies of emotional reactivity often fail to measure or show developmental differences in behavior (Baird et al. 1999, Monk et al. 2003, Thomas et al. 2001), making it difficult to constrain the interpretation of the imaging data. Recent behavioral studies of emotional reactivity suggest that adolescents have difficulty suppressing attention and actions toward emotional stimuli even when the stimuli are irrelevant to the task at hand and even when they are self-reported to be aversive (Cohen-Gilbert et al. 2014, Grose-Fifer et al. 2013). To follow-up on these findings, Dreyfuss et al. (2014) examined self-control using a measure of impulsivity (go/nogo task) in combination with cues that signal potential threat across development. Adolescents, especially males, impulsively reacted more to threat cues relative to neutral cues as compared to both children and adults (Figure 6). This adolescent-specific behavioral pattern was paralleled by greater activity in limbic cortical regions implicated in the regulation of emotional responses when correctly inhibiting a response to the social threat cues. A similar, less robust pattern was observed in the ventral striatum.
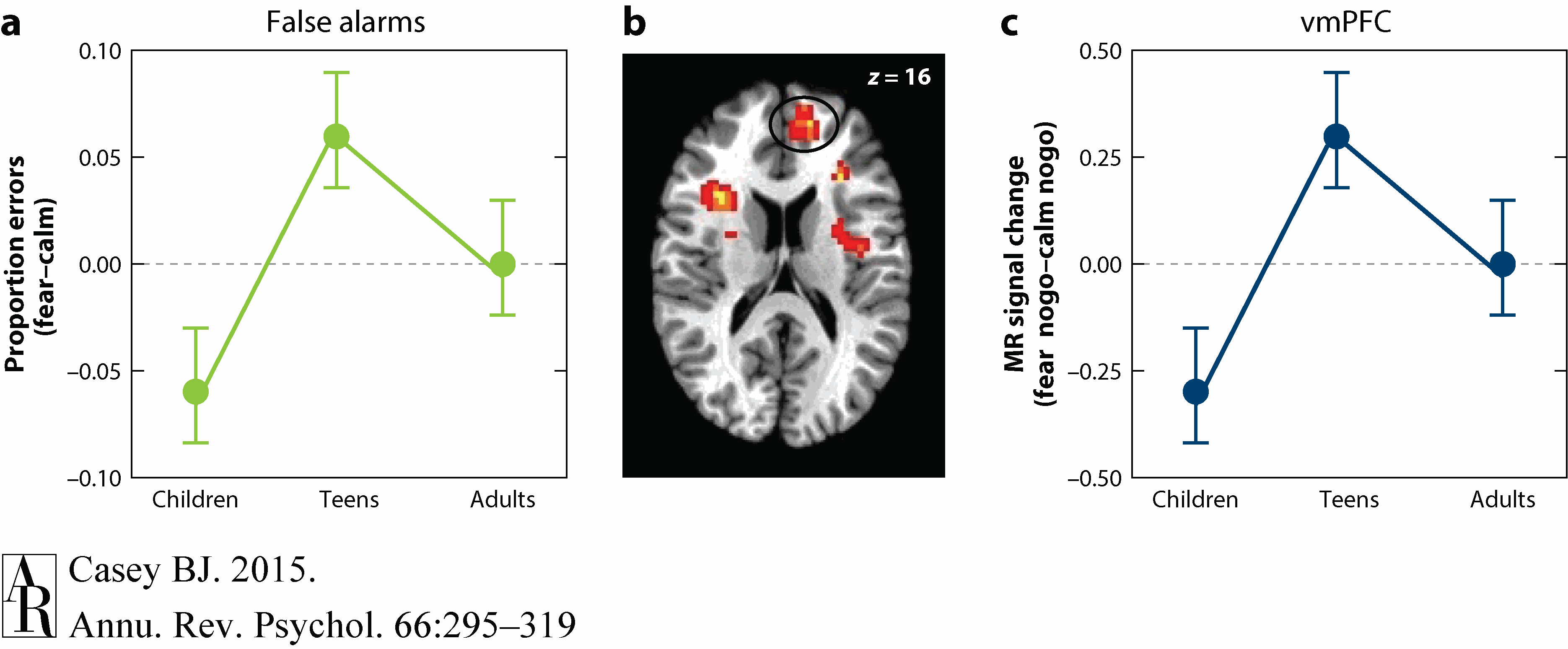
Figure 6 Dreyfuss et al. (2014) with permission from S. Karger AG, Basel.
Given adolescent-specific enhanced amygdala activity to threat cues (Hare et al. 2008) together with evidence from Stuber and colleagues (2011) showing that amygdala input to the ventral striatum facilitates motivated action, it is possible that the increased impulsivity in adolescents is due to less mature prefrontal top-down regulation of this amygdala input. However, neither activity in the ventral striatum nor in the amygdala reached significance during correctly inhibited responses in these studies. Presumably, this is due to the successful cortical suppression of this subcortical circuit on correct trials, but follow-up studies are necessary to confirm this interpretation.
Together, these findings suggest that heightened reactivity to emotional cues together with changing prefrontal cortical development may result in an imbalance in the recruitment of these regions and a breakdown in self-control. However, the previous studies relied upon human facial expressions to examine developmental differences in self-control in the presence of potential threat. Although these cues may be some of the most compelling for humans in the detection of impending danger, individuals dramatically differ across age in their experiences with them. These differential experiences may confound the interpretations of any developmental differences in response to them. An alternative approach is that of Pavlovian conditioning, which can be used across ages (developmental transitions) and across species (translation).
Cued Fear
Pavlovian conditioning involves pairing a neutral cue such as a tone with an aversive stimulus (e.g., shock). With repeated pairings, the neutral cue takes on the negative qualities of the aversive stimulus. This fear memory is extinguished when the cue is presented repeatedly without the aversive consequences. How does cued fear learning and extinction differ across development, specifically during the adolescent period when self-control appears to be diminished in the presence of motivational and emotional cues?
A recent study examined the development of fear learning and extinction in humans and mice and demonstrated the utility of using both translational and developmental transitions approaches (Pattwell et al. 2012). Children ages 5 to 11 years with a Tanner pubertal stage of 1, adolescents ages 12 to 17 years with Tanner pubertal stages of 2 to 4, and adults ages 18 to 28 years with a Tanner pubertal stage of 5 were tested in a cued fear conditioning and extinction paradigm. Yellow or blue colored squares were paired with an aversive burst of white noise during fear acquisition and then presented in isolation during fear extinction. Galvanic skin conductance responses were used as a measure of fear acquisition to the conditioned cue (colored squares). All three age groups showed equivalent fear acquisition. However, adolescents showed a diminished capacity to extinguish the fear association relative to both children and adults (Figure 7).
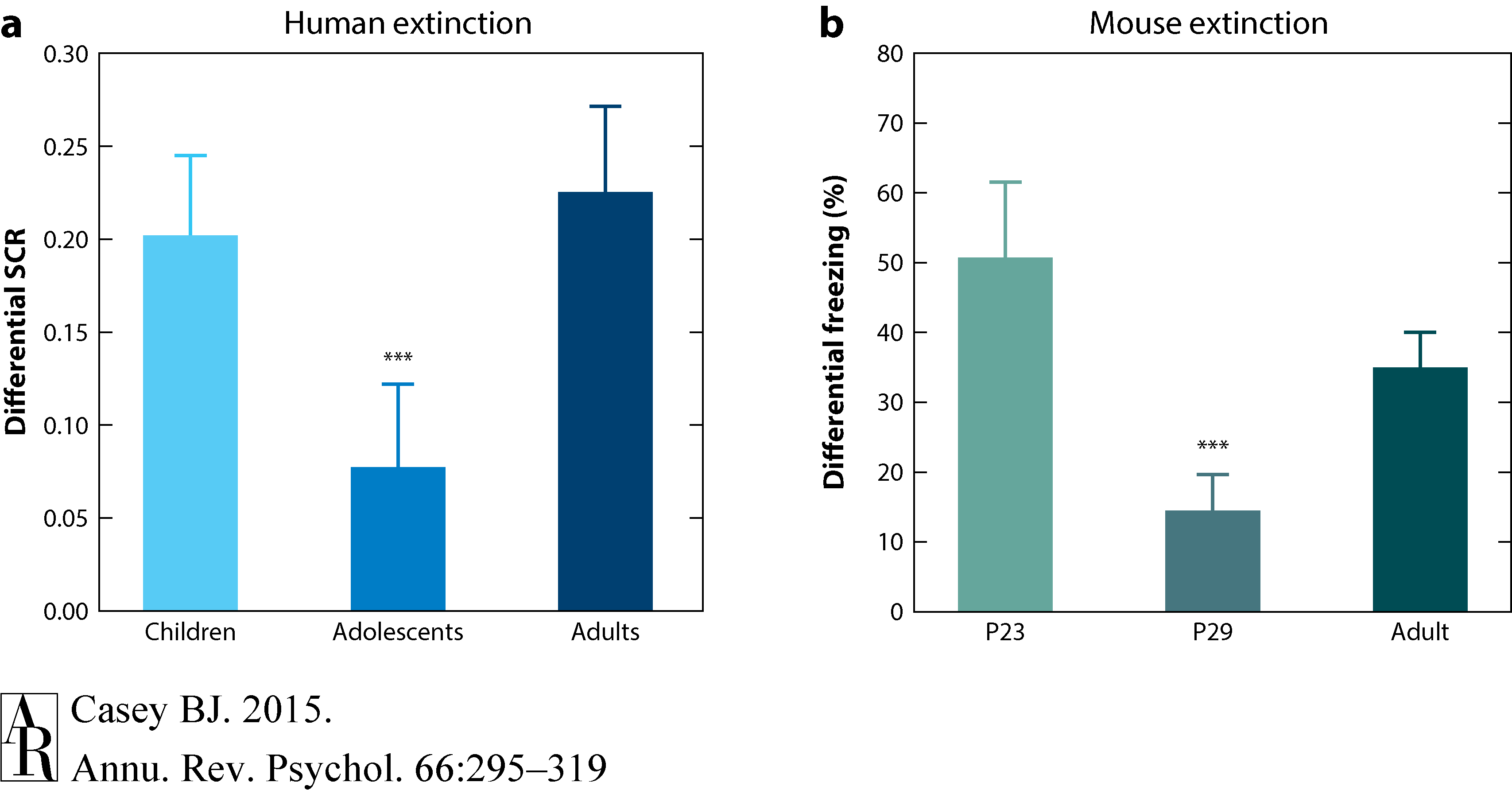
Figure 7 Pattwell et al. (2012).
A parallel test was conducted on mice once they were weaned on postnatal day 23 (P23), in early adolescence (P29), and early adulthood (P70). A neutral tone was paired with an electric foot shock, and then the amount of freezing to the tone alone was used as a measure of conditioned fear. Consistent with the findings in humans and previous findings in rats (McCallum et al. 2010), adolescent mice showed less fear extinction learning than either the preadolescent or adult mice (Figure 7). This diminished extinction learning during adolescence in mice was shown to correspond with a period of less activity and plasticity in infralimbic cortex relative to the amygdala (Pattwell et al. 2012).
These findings suggest that adolescence is a period during which cued fear associations are less easily regulated, which may in turn diminish the capacity for self-control in the presence of cues of potential threat. However, we rarely experience potential cues of threat in isolation but rather within specific contexts. Let's return to the example of a snake in the grass versus a snake in a pet store or zoo. Although a fear response is adaptive and important for self-survival in the context of a snake in the grass, a fear response in the safety of a pet store or zoo is not. Thus, contextual information plays an important role in regulating behavior in the presence of potential threats.
Contextual Fear
Unlike cued fear, contextual fear relies on the capacity to learn about threats in the spatial confines of the environment. In rodents, contextual fear learning is tested by pairing an aversive stimulus such as foot shock with a specific context and later returning the animal to that context in the absence of a cue (e.g., tone) and measuring freezing behavior. The hippocampus, through its projections to the amygdala and prefrontal cortex, mediates fear responses on the basis of whether the specific environment has been associated with safety or danger. But, does contextual fear learning change with age?
To explore this question, Pattwell and colleagues (2011, 2012) examined contextual fear conditioning in mice at the same ages as those in the previously described cued fear conditioning experiment in mice. Mice were conditioned and then tested for contextual fear the following day. Adult mice (P70) showed the typical increase in freezing behavior when placed back in the context in which they were shocked the previous day. Preadolescent mice (P23) showed the same pattern. Surprisingly, adolescent mice (P29) showed little if any fear when placed back in the conditioned context (Pattwell et al. 2011). To determine whether there was a sensitive period for suppressed expression of contextual fear, several cohorts of mice were tested in the contextual fear paradigm as shown in Figure 8. The results indicated that during a window of P29 to P39, adolescent mice show less freezing than younger animals (ages P23 to P27) or older animals (P49 to P70).
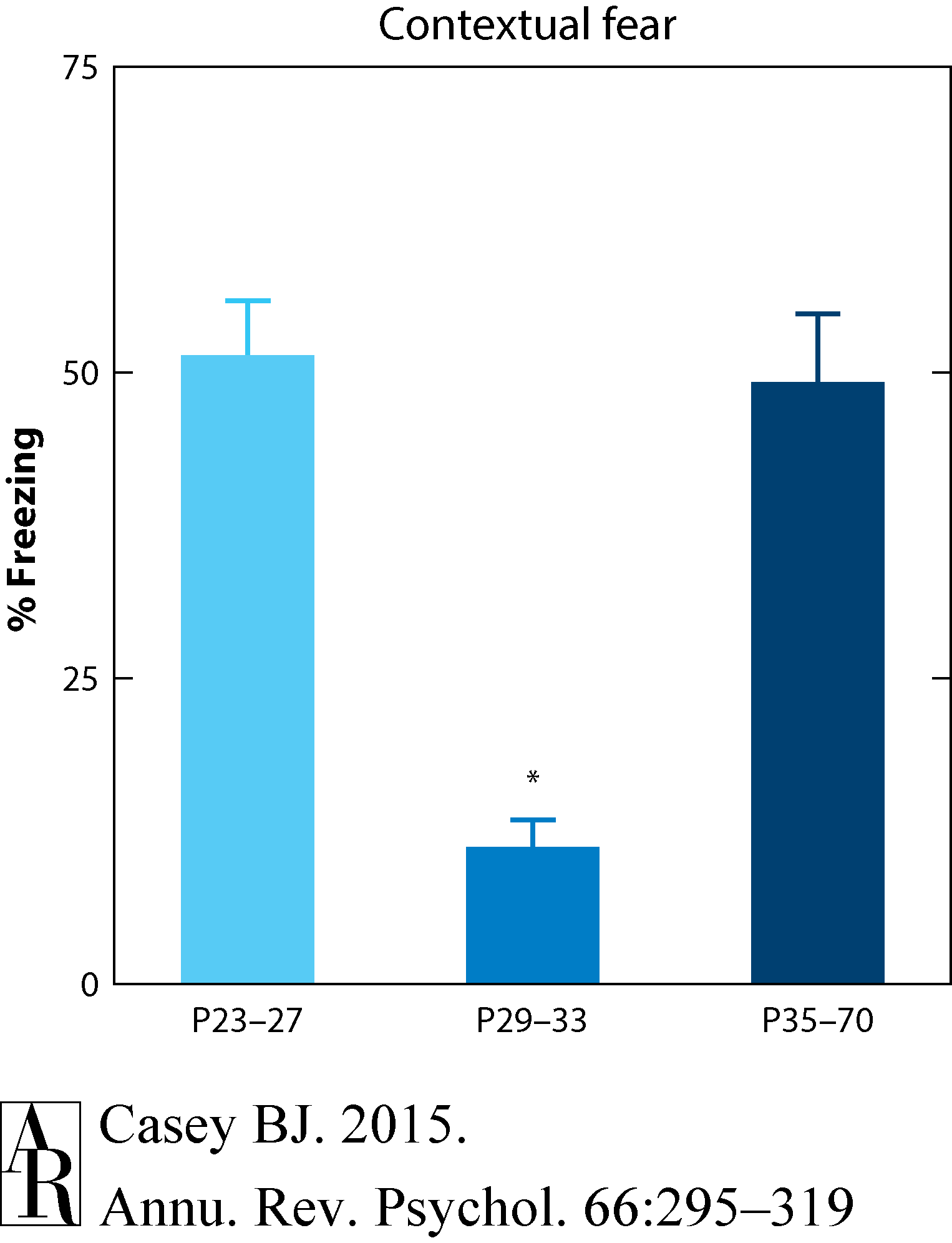
Figure 8 Pattwell et al. (2011).
What is more surprising about this adolescent-specific lack of a contextual fear response is an accidental discovery that followed nearly a month later. Specifically, mice were taken from a random cage to be used in testing new equipment for automatizing the measurement of freezing behavior. The animals were then placed in the conditioning chamber and unexpectedly froze immediately, without a shock being administered. Siobhan Pattwell, the first author of this study, went back and checked the notes on the cage, which indicated that the mice were the same mice that as adolescents showed no expression of fear after contextual fear conditioning. As adults, these mice were showing fear. In other words, the adolescents showed evidence of fear learning as adults although they showed no behavioral expression of fear immediately following contextual fear conditioning as adolescents.
To verify this finding, the investigators tested a cohort of adult mice exposed to the context and the tone during adolescence but not to the shock and saw no expression of fear (Pattwell et al. 2011). To further confirm the delay in behavioral expression of the contextual fear memory, tests were conducted on several cohorts of mice that were all conditioned at P29 but tested at P30 to P43. The behavioral expression of fear as indexed by freezing behavior was not observed until 13 days later, at P43. These results indicate a developmental window of suppressed fear.
Given that the younger mice (less than P29) were quite capable of expressing fear, a simple interpretation of a less developed prefrontal cortex driving the effects seems implausible. Instead, there appears to be a cascade of developmental changes within fear circuitry. Subsequent in vivo electrophysiological recordings suggest that the diminished contextual fear expression during adolescence is associated with blunted amygdala activity due to changes in hippocampal inputs, rather than prefrontal inputs, during contextual conditioning (Pattwell et al. 2011).
Together, these findings call into question simple dual-system or triadic models of adolescent behavior. Instead, the findings suggest a series of developmental cascades in the wiring and fine-tuning of connections within complex frontolimbic circuitry. A more plausible explanation for changes in self-control during adolescence may lie in the changing connections within intricate circuitry rather than changes in specific brain regions.
Conclusions and Implications
This synthesis of the developmental literature reveals adolescent-specific changes in the capacity for self-control that are not specific to humans but observed across species. The ability to suppress inappropriate emotions, desires, and actions in favor of alternative appropriate ones is diminished in the presence of salient environmental cues. An apparent sensitivity to environmental cues—positive and negative—leads to heightened reactivity both behaviorally and neurally in adolescents relative to both children and adults along with limited capacity to regulate these responses. In parallel, emotional contexts, especially threatening contexts, yield little behavioral response during this time. These dynamic changes in behavior are paralleled by regional changes in the strength of connections within limbic circuitry across development. How might these adolescent-specific brain and behavioral changes be adaptive or help the adolescent navigate the unique intellectual, physical, sexual, and social challenges of this transient developmental phase of life? Moreover, what are the implications for safeguarding adolescents from harm and for treating adolescents who lack the capacity to regulate their emotions and actions, leading to psychopathologies?
Why Would the Brain Be Programmed This Way?
The environment can be an extreme source of stability for the nervous systems and behaviors (Casey et al. 2010, Finlay 2007). A long evolutionary history leads to specialized mechanisms for adapting to these predicted environments. Given the importance of social status for resource-based benefits, survival, and reproduction, it would seem reasonable to assume that mechanisms have evolved to process socially relevant cues (Casey et al. 2010, Finlay 2007, Insel & Fernald 2004). At first glance, suggesting that a propensity toward motivational or emotional cues during adolescence is adaptive may seem untenable. However, a heightened activation into action by environmental cues and decreased apparent fear of novel environments during this time may facilitate evolutionarily appropriate exploratory behavior (Casey et al. 2010).
Adolescence is not unique to humans but rather is observed across mammalian species, and all adolescents must acquire the skills necessary for successfully transitioning from dependence on the parent to relative independence (Casey et al. 2010). These behaviors include increases in novelty seeking and peer interactions, which are thought to serve adaptive functions despite the risks they may have for the adolescent (Crockett & Pope 1993, Irwin & Millstein 1986, Spear 2010). Novelty seeking at this time may increase the probability of securing additional resources and forming bonds outside of the family for viability of offspring, and exploring adult privileges may increase the capacity for assuming the adult role—the ultimate goal of adolescence (Csikszentmihalyi & Larson 1987, Daly & Wilson 1987, Meschke & Silbereisen 1997).
A heightened sensitivity to socially relevant cues (rewards, peers, and threats) together with a decreased apparent fear of potentially threatening contexts may be ideal mechanisms for meeting some of the developmental challenges of adolescence. First, a pull toward incentives would help to motivate a move out of the nest or home to secure additional resources and to find new sexual partners. A heightened vigilance for detecting cues of potential threat would help the adolescent avoid immediate attack by a predator when exploring new environments. Finally, a decrease in fear of novel or potentially threatening environments would allow adolescents to leave the safety and shelter of their home to explore new environments and ultimately establish a new home. It is probably highly adaptive for adolescents not to express fear at this time but to retain memories for which contexts are potentially threatening to avoid building their nest or home in that environment. The combination of emotionality and novelty seeking may have evolved for good reasons but in today's society serves less of an adaptive purpose, as evidenced by the high mortality rates in adolescents, consistent with adolescents putting themselves in harm's way. So although adolescence is often viewed as one big roller coaster ride of thrills, it seems more plausible that this period is one of real fears as well as thrills that together have led to the survival of the fittest and, ultimately, of the species.
Implications for Mental Health
Although this review focuses on typical development of self-control in adolescents, the prevalence of psychopathology in adolescents is staggering, with one in five suffering from a mental illness. What have we learned about typical brain and behavior development in adolescence that may help us understand the increased risk for psychopathology?
Perhaps one of the first psychopathologies that comes to mind is that of substance abuse, given that adolescence is a time of increased experimentation with and use of illicit drugs and alcohol. Earlier substance use is a reliable predictor of later dependence and addiction (Grant & Dawson 1997). Alcohol and marijuana, the leading substances of choice for teens, have reinforcing properties and impact the dopamine system (Bjork et al. 2008, Hardin & Ernst 2009). Thus, these substances may exacerbate an already heightened response in the dopamine-rich region of the ventral striatum, leading to strengthening of the reinforcing properties of the drug. Specifically, elevated activity of the ventral striatum during a time when prefrontal control circuitry is still immature may result in an exacerbated imbalance among these regions and a reduced capacity to regulate use and abuse-related behaviors (Casey & Jones 2010).
A second significant psychopathology is that of the anxiety disorders, the most common of the psychopathologies in young people, affecting as many as 1 in 10. Although heightened emotionality is typical for healthy adolescents, failure to suppress emotional responses over time when there is no impending threat can become pathological. Imaging studies of individuals with anxiety symptoms and disorders show enhanced amygdala activity to repeated presentation of empty threat (e.g., fearful expressions) and less frontoamygdala circuit connectivity (Guyer et al. 2008, Hare et al. 2008, Monk et al. 2003, Thomas et al. 2001), which together may lead to symptoms and ultimately the diagnosis of anxiety. The most common form of behavioral treatment for anxiety involves cognitive-based exposure therapy that identifies the source of the anxiety and desensitizes the individual to that fear. The desensitization process builds on basic principles of fear extinction. Although there has been growing interest in fear extinction learning because of its clinical relevance to the treatment of anxiety disorders (Anderson & Insel 2006), these therapies have potential limitations. Only half of those individuals with anxiety respond to these treatments (Walkup et al. 2008), and the work highlighted in this review showed diminished fear extinction during adolescence in rodents and humans (McCallum et al. 2010, Pattwell et al. 2012), which suggests that exposure-based therapies may have less efficacy during this specific developmental period.
No study to date has been designed to directly test the hypothesis for differences in the efficacy of exposure therapy in anxious patients across age. However, as a proof of concept, response rates for cognitive behavioral therapy across age, based on published clinical trials, were examined (Davidson et al. 2004, Walkup et al. 2008). This pilot study showed a trend for reduced treatment efficacy of cognitive behavioral therapy in adolescents relative to both children and adults (Drysdale et al. 2013) (Figure 9). This work illustrates the power of a developmental transitional and translational approach for uncovering patterns of potential clinical relevance for targeted novel intervention.
Figure 9 Drysdale et al. (2013) with permission from Elsevier.]Download Full-ResolutionDownload PPT
Implications for Law and Policy
The potential influence of adolescent behavioral and brain science on law and public policy has become increasingly evident with landmark decisions regarding the culpability of juveniles by the US Supreme Court over the past decade (Cohen & Casey 2014). Since 2005, the court majority decisions first abolished the death penalty, then life without parole for crimes other than homicide, and then (in 2012) a mandatory life sentence for any crime. Developmental science on the increased impulsivity and risk taking of adolescence relative to adults was referenced in all of these cases, with explicit reference to neuroscience occurring only in recent years (Scott & Steinberg 2008, Steinberg 2009). Brain science contributes to an understanding of why adolescents engage in the behaviors that they do. Such evidence does not imply that juveniles should not be accountable for their crimes but rather that diminished responsibility should be considered in determination of the punishment for these crimes.
If we look at the 2012 US Supreme Court majority decision on Miller v. Alabama and Jackson v. Hobbs to abolish a mandatory life sentence, we see several similarities in the crimes. First, the crimes were both murders that took place in emotionally charged situations. Second, the crimes were committed with peers. Third, both defendants were 14 years of age at the time of the crimes. Our review of the self-control literature suggests that in the heat of the moment, under potential threat and in the presence of peers, emotional centers of the adolescent brain may hijack less mature prefrontal control circuits, leading to reckless behaviors (Cohen & Casey 2014). Thus, under the Eighth Amendment, subjecting juveniles to the same punishments as adults may be considered cruel and unusual punishment. Moreover, by definition, adolescence is a transient period of development during which the individual must learn to meet new challenges in order to become an independent adult in society. Locking up juveniles results in limited opportunity for developing social skills and regulating their own emotions and actions, which may hinder this development (Dmitrieva et al. 2012).
Developmental brain imaging data together with behavioral data that assess capacities relevant to criminal responsibility under circumstances in which individuals often commit antisocial acts (e.g., emotional arousal, peer presence, etc.) have potentially important implications for the legal regulation of juvenile crime. First, evidence that immature brain functioning in adolescence influences judgment, decision making, risk taking, and criminal behavior may bear on important legal questions regarding culpability and punishment of adolescents and provide new insights for minimum ages for legal policies. Second, this work may inform and improve policies on the treatment of adolescents to promote rehabilitation through intervention, bolstering healthy adolescent development and thereby reducing recidivism (Bonnie & Scott 2013, Natl. Res. Counc. 2013).
Summary
Thinking of adolescence as one big roller coaster ride of thrills in the face of potential danger is one way of characterizing this developmental phase. However, this characterization does not capture the exquisite and intricate transformations of brain and behavior during this developmental phase (Helfinstein & Casey 2014). Changes in self-control during adolescence parallel a series of developmental cascades in the wiring and fine-tuning of connections within complex subcortical and cortical prefrontal and limbic circuitries. These adolescent-specific changes reflect both evolutionarily based biological constraints and unique intellectual, physical, sexual, and social experiences. Having sufficient time and space to freely explore and experiment may enhance the formation of the adolescent's self-identity (Erikson 1968) and lead to healthy development into a socially functioning adult.
SUMMARY POINTS
1. | Adolescence is a normal and transient stage of development that reflects the transition from childhood to adulthood beginning around the onset of puberty and ending with relative independence from the parent. |
2. | A heightened activation into action by environmental cues and decreased apparent fear of novel environments during adolescence may facilitate evolutionarily appropriate exploratory behavior. |
3. | Changes in self-control during adolescence consist of rewiring and fine-tuning of connections within complex subcortical and cortical prefrontal and limbic circuitry. |
4. | The brain is sculpted by evolutionarily based biological constraints and experiences as it adapts to the unique intellectual, physical, sexual, and social challenges of adolescence. |
5. | Developmental transitional and translational approaches to understanding adolescence have uncovered patterns of clinical relevance for targeting novel interventions. |
6. | Work on the adolescent brain and behavior may inform and improve policies on the treatment of juveniles through intervention, bolstering healthy development of adolescents into prosocial adults. |
FUTURE ISSUES
1. | Translational studies across species will help to delineate mechanistic changes in cortical and subcortical circuitry that underlie changes in self-control with development. |
2. | The future holds immense opportunity and an obligation to move basic research more quickly from the laboratory to the clinic in the prevention and treatment of mental illness in young people as we target processes of change in the capacity for self-regulation (Casey et al. 2014). |
3. | The collection of developmental brain imaging data together with behavioral data that assess self-control capacities relevant to criminal responsibility may inform and improve policies on the treatment of adolescents, promote rehabilitation, bolster healthy adolescent development, and ultimately reduce recidivism (Cohen & Casey 2014). |